4 Chapter 4: Evolution and Human Adaptation
Amy Rector
By the end of this chapter, you should be able to:
- Discuss differing perspectives about how the human species descended from a primate ancestor.
- Explain the process of natural selection.
- Examine and correct several misconceptions about human evolution.
- Discuss Darwin’s theory and contributions to our understanding of evolution.
- Explain and identify the purpose of both DNA replication and protein synthesis.
- Describe how specific patterns of human adaptations and adjustments are correlated to natural selection processes.
The Science of Who We Are
If you were to do an internet search for the word “science,” you would come up with a variety of definitions that all center around a few main ideas: science is knowledge or a system of knowledge that reflects truth about the natural world. Because this truth is obtained and tested through the scientific method, science is described as unbiased. Observations based on the scientific method are assumed to be objective and real. At the same time, scientists themselves are also thought of as unbiased, objective, and in search of truth and real observations about the natural world.
The scientific method is based on a series of steps that include observing a pattern or occurrence, formulating a hypothesis about that pattern or occurrence, and collecting data to test the validity of that hypothesis. Each step is expected to be unbiased, so that data-driven conclusions based on tested hypotheses can be safely interpreted as valid, or real.
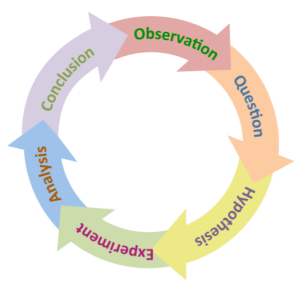
But what if the scientists who are observing the natural world, looking for patterns or occurrences, and then formulating hypotheses to test are themselves biased? Anthropology teaches us that culture, beliefs, and worldviews are learned by humans as we grow up; who we are and what we believe are the products of enculturation, or living, observing, and learning in groups. Each one of us – and how we look at the world – is the synthesis of where, when, how, and with whom we grew up. Ultimately, this means that we are all biased by our backgrounds. Scientists who are attempting to identify and explain patterns in the natural world must be extremely vigilant about identifying and examining their own biases, or the validity of their hypotheses, data, and conclusions might be at risk.
Read this comic by The Oatmeal about core beliefs, and then think about your own core beliefs. How do your core beliefs influence how you see the world? How do they influence how you ask questions about the world?
When scientists don’t recognize their own core beliefs and biases – and how they might influence their scientific inquiry and interpretations – the results and conclusions of scientific studies can be biased in pretty significant ways. In some cases, scientific studies are not only based on biased views of the world, they are used to validate biases because they confirm what is expected based on the core beliefs of the scientist. This is why it is critical for scientists to examine their own core beliefs and biases as they design studies and interpret data – especially if the scientific inquiry is about humans. Early scholars of human biology and evolution were deeply plagued by their own core beliefs and biases, usually related to the barriers created by religious beliefs and/or racist conceptions of the world. It is useful to explore how the scientific exploration of humans and our closest relatives has changed over time, and how these biases have shaped our understanding of our own humanity in different ways.
Early Evolutionary Scholars
Some of the earliest evolutionary scholars were Muslim scholars who are often not included in the western scientific canon because their works were not published in English. It is often speculated, however, that later scholars had access to these works and may have borrowed heavily from them. Al-Jahiz was born in 776 in what is now Iraq, and was the first scholar to propose a theory of evolutionary biology in his Book of Animals (Figure 2). This book also described a process by which evolution might occur that is very similar to what Charles Darwin would later describe as “natural selection.”
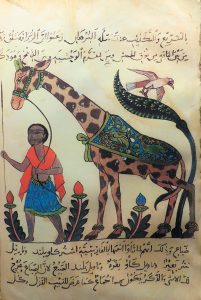
Nasir ad-Din Tusi was a 13th century Persian Muslim scholar who wrote the book Akhlaq Nasiri (Nasirean Ethics). While this work is best known for its philosophy, math, and theology themes, Tusi also touches on evolutionary ideas around the origins of humans and the evolution and variation of species more than 600 years before Darwin did. Ibn Khaldun was born in 1332 in what is now known as Tunisia, and is broadly recognized for his efforts in social sciences, sociology, and history. In 1377, he wrote Al-Muqaddimah (Prolegomena), and specifically hypothesized that all species evolve, and that humans evolved from an ape or monkey ancestor. These Muslim scholars, like Christian scholars both before and after the Enlightenment, struggled with religious doctrines regarding evolution and the origins of humans and how their scientific observations did nor did not reflect these beliefs.
In the 17th and 18th centuries, European Enlightenment scientists focused on exploration and the pursuit of knowledge through reasoning and science, and were beginning to look at the world around them more systematically. Despite a general reluctance to consider humans as belonging to the same category as animals, around 1700 the English anatomist Edward Tyson published the first known anatomical study of the animal we now call a chimpanzee. HIs work demonstrated that it was physically more similar to us than to any other creature known. He even counted up its similarities: the chimpanzee resembled humans in 48 ways, but monkeys in only 34 ways (see Figure 3).
Systema Naturae was a series of books by Swedish naturalist Carl Linneaus that attempted to illustrate and organize all the known species in the natural world. The tenth edition of Systema Naturae , published in 1758, is considered to be the first use of modern taxonomy – or how we organize and name groups of species based on how they look and how they are related to each other. We call this organization the hierarchical classification scheme, and it includes taxonomic groups like Kingdoms, Orders, and Species. Where animal species had traditionally been linearly conceptualized in terms of how similar to humans they are—forming a “Great Chain of Being”—Linnaeus identified a distinctly different pattern. In so doing, Linnaeus found that warm-blooded, hairy, lactating vertebrates formed a natural group that he named “Mammalia” in 1758 (in contrast to, say, fish or birds). Within that group was a cluster of species he called “Primates,” and among them, according to our physical features, was our own species, which he named Homo sapiens. These physical correspondences among diverse kinds of creatures later came to be known as homology. But why did such a pattern of nested similarities exist, and what did it mean? Linneaus grew up in Sweden in the 18th century, which meant that his world view was deeply shaped by the idea that European populations and cultures were the most advanced in the world. This core belief influenced his scientific inquiry. Linnaeus was the first naturalist to include humans as part of the animal kingdom, but his core beliefs influenced hundreds of years of scientific thought about how humans and human variation fit into the natural world.
A question that scientists also grappled with was that of adaptation and biogeography, and what fossil discoveries meant. Why were different species located in different environments? What influenced where you might find some species, and not others? These were some of the questions that dominated the field of natural history by the beginning of the 1800s. But of course the big questions of the day weren’t even about why some animals were extinct and others were only found in certain places at all but, rather, about the biopolitics of slavery. Were all people of one stock, the descendants of Christianity’s Adam and Eve? That would seem to afford a moral argument against treating some people as property, if we are all share the same ancestors. This position, however, required the development of a biological theory to explain how Adam and Eve’s descendants could have morphed into the diverse peoples of the world. In other words, if you imagined Adam and Eve to be White, then how did Black people arise? (Or vice versa.) This position, that all humans are descended from one origin, was known as monogenism, was biblical, socially progressive, and generated the earliest modern evolutionary theories—intended to explain the naturalistic production of variation in populations, or what we would now call evolution.
Others believed that Africans and Europeans shared no common ancestry at all, being the products of separate creations. Perhaps in Adam and Eve, the Bible was merely recounting one story of creation, but the peoples of the rest of the world were fundamentally and unalterably different and had always been so. This position, known as polygenism, was attractive to those looking to rationalize slavery as well as to radical intellectuals who did not feel constrained by biblical literalism.
Darwin’s Mechanism of Evolution
The publication of The Origin of Species by Charles Darwin in 1859 became an intellectual flash point in European intellectual life. It was focused on a significantly narrow point: Where do new species, adapted to their surroundings, come from? Charles Darwin had come to think about the origin of species upon returning from a long voyage around the world in the early 1830s on the H.M.S. Beagle. In South America, Darwin had observed that the unusual species he saw alive there were very similar to the unusual extinct animals in the same area. This suggested some sort of historical continuity between them—descent with modification, he called it. The problem was how to make sense historically of the particular adaptations that differentiate species. The engine of adaptation, Darwin realized, was competition. This did not necessarily entail face-to-face competition but simply the fact that not all members of a species are equally likely to survive and breed. Which ones are more likely? The ones that randomly are a bit more in sync with their environment. Those creatures will disproportionately thrive and breed, and the next generation of the species will come to look just a bit more like them, on the average. The core of Darwin’s thought is thus a two-step process: the random generation of variation, and the nonrandom process by which the environment subtly favors organisms with certain features to thrive and breed.
Darwin called this principle natural selection and planned to write a long book about it someday. But in 1858 he received a manuscript from a fellow naturalist, Alfred Russel Wallace, who had come up with quite similar ideas to his own while working in the Malay archipelago. Darwin’s friend, the geologist Charles Lyell, had papers by Darwin and Wallace read into the record, The Transactions of the Linnaean Society, July 1, 1858, so they could share credit for the discovery, and Darwin set about to publish the work he had done on natural selection. The result was called On the Origin of Species by Means of Natural Selection, or the Preservation of Favoured Races in the Struggle for Life, published on November 24, 1859.
Darwin’s central thesis was that the differences among breeds or strains or varieties of animals and plants were the same kinds of differences that exist between species, only smaller and formed over shorter periods of time. The origin of new species lay in the long-term biases of survival and reproduction in older species. The result was a convincing naturalistic explanation for adaptation. Moreover, it finally explained the nested pattern of similarities among species that Linnaeus had discovered a century earlier but couldn’t explain. Those nested patterns were the legacy of common ancestries; they were literally family resemblances.
Darwin was especially careful to omit any discussion of people from his book. He wanted the discussion to be about the general process; consequently he wrote just a single line, near the end, about people: “Light will be thrown on the origin of man and his history” (Darwin 1859, 488). He was willing to acknowledge the possibility that life had “been originally breathed into a few forms or into one,” but he was satisfied with having described the mechanism by which adaptive change has taken place in the organic world since then—in parallel with Isaac Newton, who famously refused to speculate on where gravity came from, focusing instead only on how it works.
Within the academy, there was not too much reaction against the proposition that humans had descended with modification from an ape stock, and had then differentiated from that stock over the eons as a result of the differential preservation of favorable variations. The heart of Darwinism as applied to humans is simply ape ancestry and adaptive divergence.
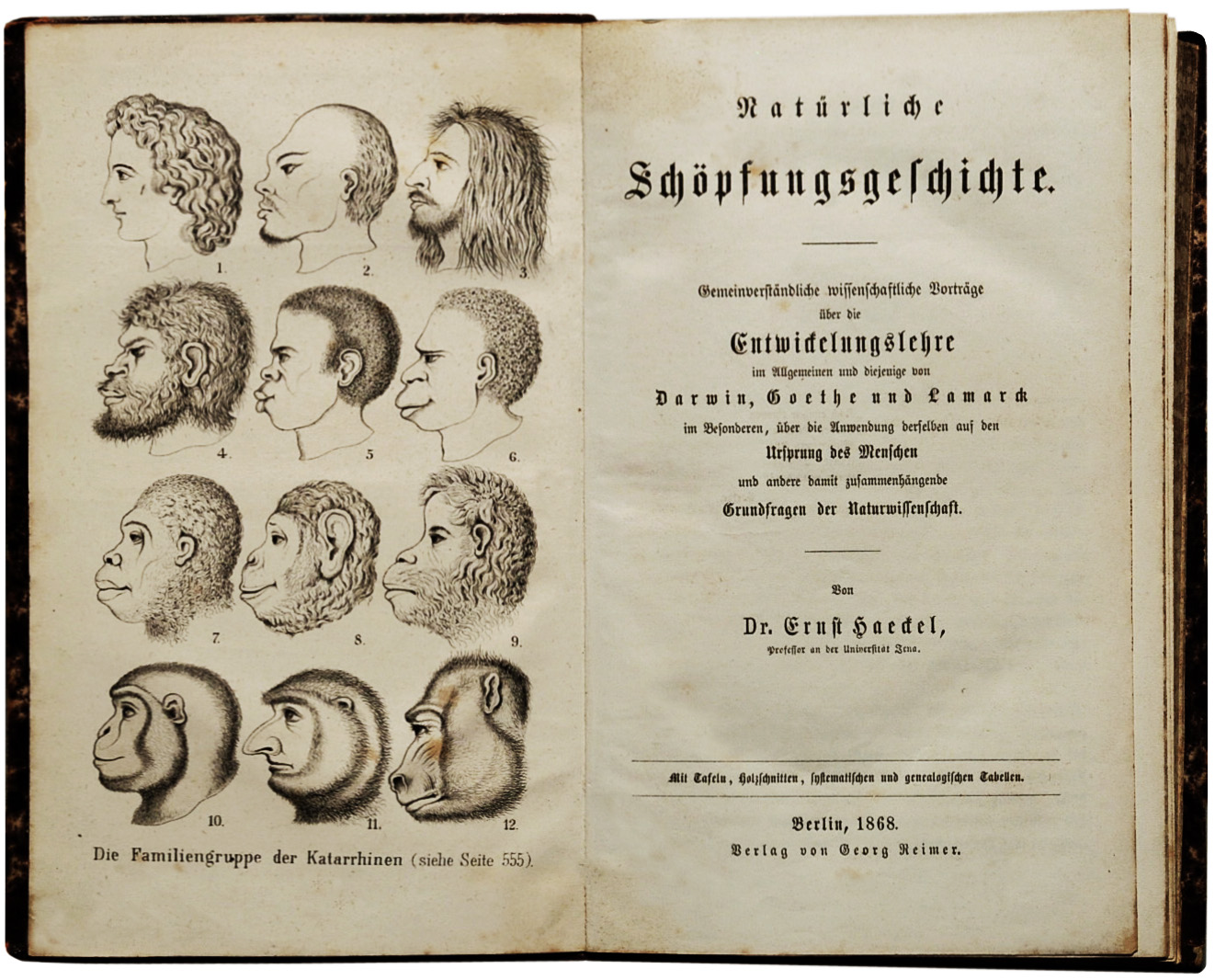
But the early Darwinians were faced with a dilemma—in 1860, there was no fossil evidence linking humans to apes. The German biologist Ernst Haeckel solved this problem by fatefully arguing that we don’t need a fossil record to link us to the apes, because Europeans are linked to the apes through the non-White peoples of the world. He envisioned 12 different species of living peoples, each at different distances from the apes, thus sacrificing the full humanity of most people on the altar of Darwinism (see Figure 4). Scientists of the 1860s thought the full humanity of Africans was less important than evolution. Today that position is morally repugnant. While Darwin and his English colleagues did not agree with these details, they nevertheless saw Haeckel as an ally in the broader struggle to get evolution accepted. With hindsight, we can judge this to be a morally questionable decision: Today we would hopefully universally consider the full humanity of Africans to be more important than whether humans are descended from apes, and thoroughly repudiate anyone who denied it.
Post-Darwinian Theories and Disputes
The immediate theoretical weakness of Darwinism lay in its reliance upon a pool of undirected variation for nature to select from. Darwin fell back on a principle developed by Lamarck known as the “inheritance of acquired characteristics” or “use and disuse of organs.” Here, whatever attributes you develop over the course of your life—muscles, a tan, compassion, bad breath—can be stably passed on to your children, somehow. That way, variation can be reintroduced every generation, by virtue of this new pool of acquired features.
The entire problem was rendered moot with the discovery in 1900 of Gregor Mendel’s work on heredity in peas from 35 years earlier. Mendel showed that heredity didn’t actually work like the blending of paints. When you isolated particular traits, you saw that offspring were not midway between their parents; rather, they were like one or the other parent. The offspring of a plant with green peas and one with yellow peas was green, not chartreuse. The offspring of a plant with wrinkled peas and one with round peas was round, not wrinkly-round. This suggested, rather, that heredity worked like interacting particles that came into new combinations but fundamentally retained their structural integrity every generation. Unlike paints, you could indeed recover the original variants under this model; variation wasn’t lost every generation.
Mendelian genetics soon created new problems for Darwinism, however. The new geneticists were focused on discrete binary states of existence, like Mendel’s peas: green/yellow, wrinkled/round, tall/short, in experimental populations. But the old Darwinian naturalists were working with quantitative variations in real populations—many of them intermediate, not extreme, in form. So, the Mendelians had a robust theory of heredity that had difficulty explaining natural patterns of variation, and the Darwinians had a robust theory of biological change that had difficulty accommodating discontinuous variation. One solution might be to reconceptualize all variation as fundamentally binary; the American geneticist Charles Davenport, for example, argued with considerable success that there were two kinds of people—smart and stupid—and that the stupid people simply had the allele for “feeblemindedness.” This actually had a major and regrettable impact on American science and social policy in the 1920s.
A better solution came with the invention of population genetics, in works published around 1930 by the British geneticists Ronald Fisher and J. B. S. Haldane and the American geneticist Sewall Wright. In this model, a gene has small but cumulative effects. If we reduce a body to its genetic composition or genotype, and we reduce a species to its cumulative genetic composition, or gene pool, we can mathematically model the ways in which the gene pool can be transformed. There are rather few ways to accomplish it, and each has characteristic and predictable effects.
This became the first part of the Synthetic Theory of Evolution, the extension of Mendelian genetics to population genetics and the formal mathematical study of how gene pools may be transformed through time. The second part involved the study of how species diversify in addition to simply changing, and it entailed integrating speciation and geography in the story of how animal species have come to be.
By the 1960s, then, biologists had a robust theory to explain the history of life. Genetic or genotypic changes (known to be encoded in molecules of DNA) cause changes in the physical appearance or phenotype. The environment sorts out these changes, and their proportion within a species rises or falls with the nature and stringency of the environment. Selection could now be reduced to the favoring of certain genotypes over alternatives, which can make populations genetically adaptively different from one another. Genetic drift, or stochastic (random) changes to the gene pool, makes populations genetically different from one another non-adaptively—that is to say, in ways that don’t track the environment. The genetic contact of populations, or gene flow, makes populations more similar to one another. Disrupting gene flow acts to divide gene pools, which is in turn stabilized by the development of reproductive barriers between the populations. These processes can be directly studied within living species and can be extrapolated and can adequately explain the differences we find among species.
Further work in population genetics revealed that most of a species’ genome’s DNA falls between genes and does not actually code for anything. Consequently, mutations that occur to most of the DNA do not have discernible effects on the body and are thus non-adaptive. Only a small bit of the DNA, it seems, actually builds the organism and encodes its adaptations; and even today, the processes by which it does so are vaguely understood.
CELLS AND MOLECULES
Molecules of Life
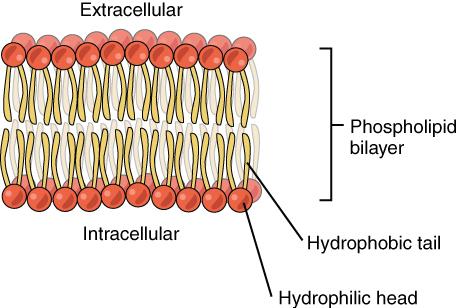
Organisms are composed of four basic types of molecules that are essential for cell structure and function: proteins, lipids, carbohydrates, and nucleic acids. Proteins are strings of amino acids that are often folded into complex 3-D shapes. The structure of lipids can be described as having a hydrophilic (water-loving) head and a hydrophobic (water-repelling) tail (Figure 5). When lipids are chained together, they form more-complex molecules called fats and triglycerides. Carbohydrates are composed of carbon and hydrogen atoms that can be broken down to supply energy for an organism. Lastly, nucleic acids carry genetic information about a living organism.
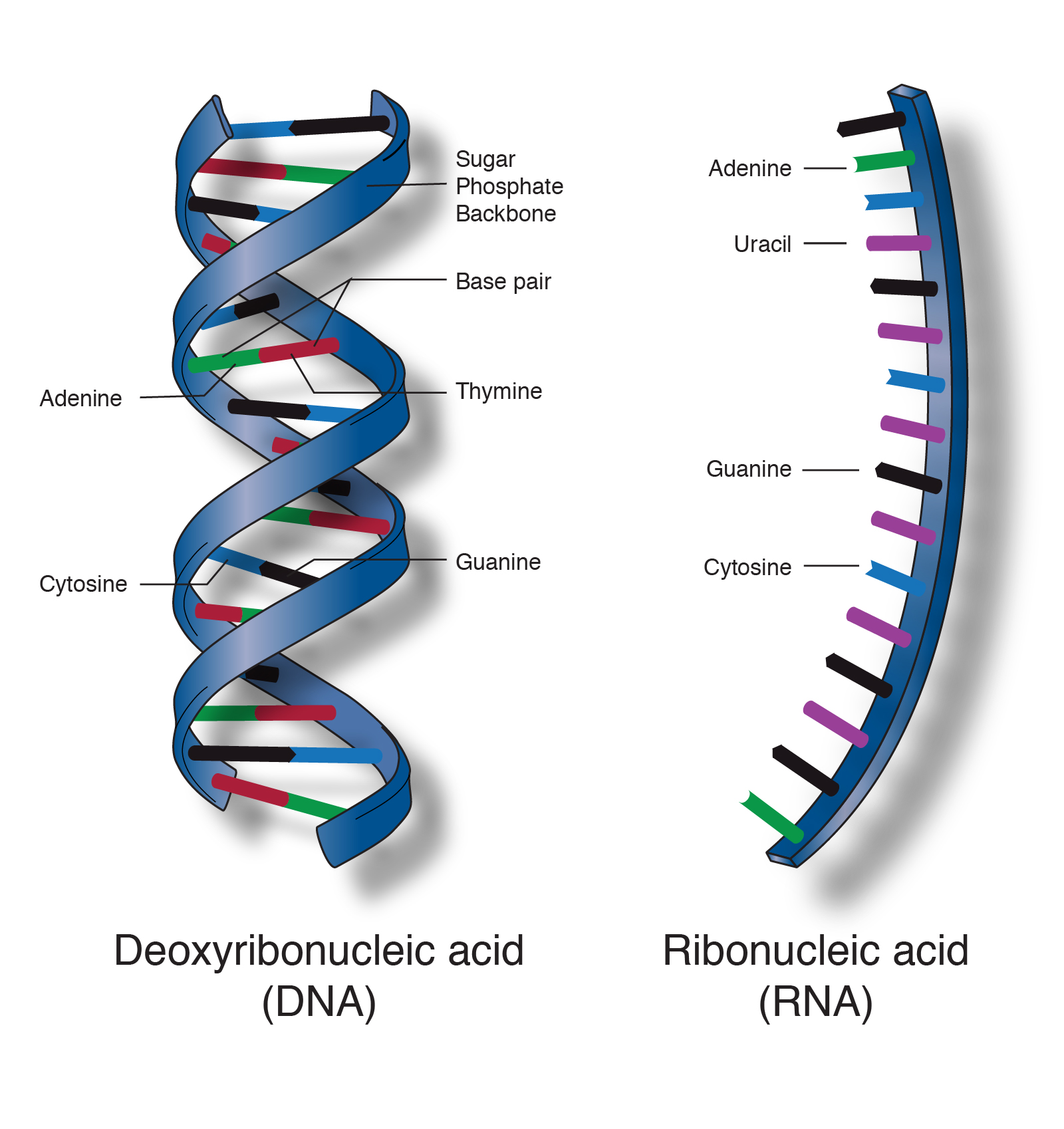
Probably the most familiar nucleic acid is deoxyribonucleic acid (DNA). DNA comprises a sugar phosphate backbone and nucleotides (Figure 6). Anthropologists can analyze sequences of DNA nucleotides and determine how different organisms are related to each other, since they all have their own unique DNA genetic code. In the case of humans, forensic scientists can identify individuals by analyzing 20 different short DNA sequences known as “CODIS Core Loci.” Another nucleic acid is called ribonucleic acid (RNA). One type of RNA molecule is responsible for chaining amino acids together in order to build proteins.
DNA Structure
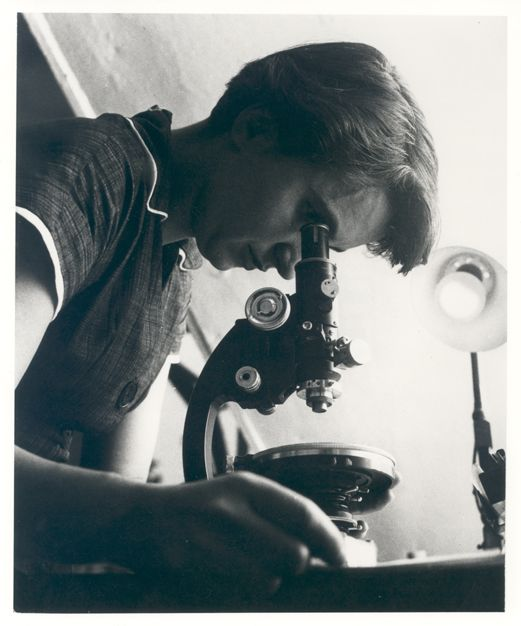
The 1953 discovery of the molecular structure of DNA was one of the greatest scientific achievements of all time. Using X-ray crystallography, Rosalind Franklin (Figure 7) provided the image that clearly showed the double helix shape of DNA. However, due to a great deal of controversy, Franklin’s colleague and outside associates received greater publicity for the discovery. In 1962, James Watson, Francis Crick, and Maurice Wilkins received a Nobel Prize for developing a biochemical model of DNA. Unfortunately, Rosalind Franklin had passed away in 1958 from ovarian cancer. In current times, Franklin’s important contribution and her reputation as a skilled scientist are widely acknowledged.
The double helix shape of DNA can be described as a twisted ladder (refer back to Figure 6). More specifically, DNA is a double-stranded molecule with its two strands oriented in opposite directions (i.e., antiparallel). Each strand is composed of nucleotides with a sugar phosphate backbone. There are four different types of DNA nucleotides: adenine (A), thymine (T), cytosine (C), and guanine (G). The two DNA strands are held together by nucleotide base pairs, which have chemical bonding rules. The complementary base-pairing rules are as follows: A and T bond with each other, while C and G form a bond. The chemical bonds between A—T and C—G are formed by “weak” hydrogen atom interactions, which means the two strands can be easily separated. A DNA sequence is the order of nucleotide bases (A, T, G, C) along only one DNA strand. If one DNA strand has the sequence CATGCT, then the other strand will have a complementary sequence GTACGA. This is an example of a short DNA sequence. In reality, there are approximately three billion DNA base pairs in human cells.
DNA Is Highly Organized Within the Nucleus
If you removed the DNA from a single human cell and stretched it out completely, it would measure approximately two meters (about 6.5 feet). Therefore, DNA molecules must be compactly organized in the nucleus. To achieve this, the double helix configuration of DNA undergoes coiling. An analogy would be twisting a string until coils are formed and then continuing to twist so that secondary coils are formed, and so on. To assist with coiling, DNA is first wrapped around proteins called histones. This creates a complex called chromatin, which resembles “beads on a string” (Figure 8). Next, chromatin is further coiled into a chromosome. Another important feature of DNA is that chromosomes can be altered from tightly coiled (chromatin) to loosely coiled (euchromatin). Most of the time, chromosomes in the nucleus remain in a euchromatin state so that DNA sequences are accessible for regulatory processes to occur.
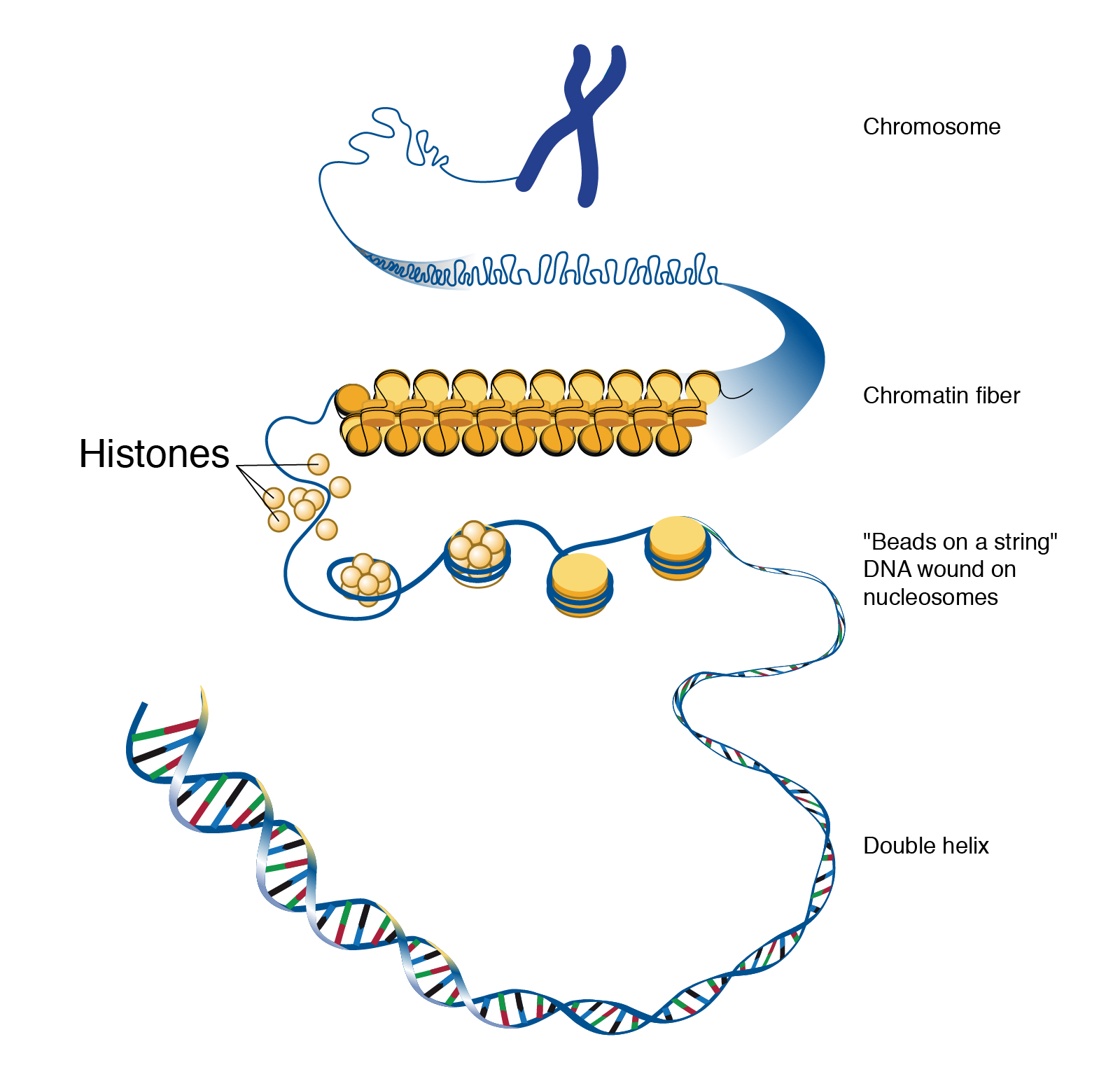
Human body cells typically have 23 pairs of chromosomes, for a total of 46 chromosomes in each cell’s nucleus (Figure 9). An interesting fact is that the number of chromosomes an organism possesses varies, and this figure is not dependent upon the size or complexity of the organism. For instance, chimpanzees have a total of 48 chromosomes, while hermit crabs have 254. Chromosomes also have a distinct physical structure, including centromeres (the “centers”) and telomeres (the ends) (Figure 10). Because of centromeres, chromosomes are described as having two different “arms,” where one arm is long and the other is shorter. Centromeres play an important role during cell division, which will be discussed in the next section. Telomeres are located at the ends of chromosomes and they help protect the chromosomes from degradation after every round of cell division. However, our telomeres become shorter as we age, and if chromosome telomeres become too short, then the cell will stop dividing. Therefore, the link between the regulation of telomere length and cellular aging is of great interest to researchers.
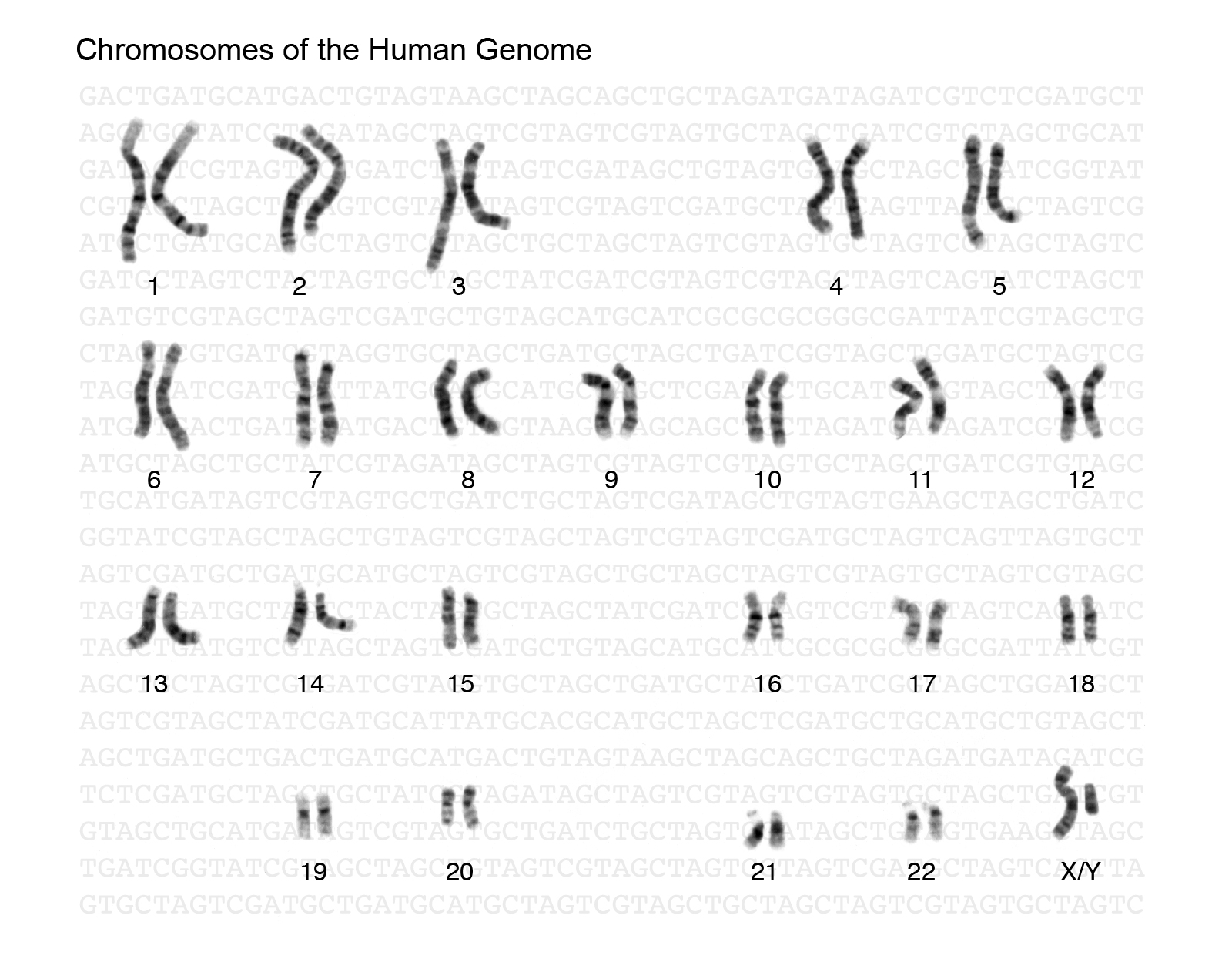
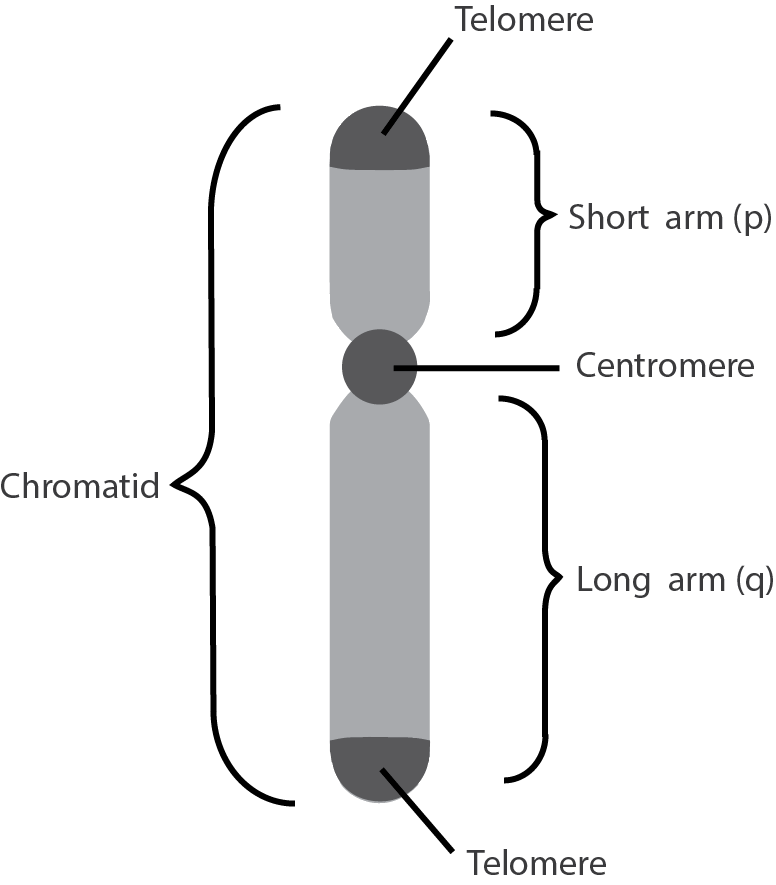
DNA Replication and Cell Division
For life to continue and flourish, cells must be able to divide. Tissue growth and cellular damage repair are also necessary to maintain an organism throughout its life. All these rely on the dynamic processes of DNA replication and the cell cycle. The mechanisms highlighted in this section are tightly regulated and represent only part of the life cycle of a cell.
DNA Replication
DNA replication is the process by which new DNA is copied from an original DNA template. It is one phase of the highly coordinated cell cycle and requires a variety of enzymes with special functions. Specifically, enzymes carry out the structural and high-energy reactions associated with replicating a double helical molecule. The creation of a complementary DNA strand from a template strand is described as semi-conservative replication. The result of semi-conservative replication is two separate double-stranded DNA molecules, each of which is composed of an original “parent” template strand and a newly synthesized “daughter” DNA strand.
DNA replication progresses in three steps: initiation, elongation, and termination. Initiation denotes the start of DNA replication by recruiting enzymes to specific sites along the DNA sequence. For example, the double helix of DNA presents structural challenges for replication, so an initiator enzyme, called helicase, “unwinds” DNA by breaking the hydrogen bonds between the two parent strands. The unraveling of the helix into two separated strands creates a fork, which is the active site of replication machinery (Figure 3.17). Once both strands are separated, the parent template strands are exposed, meaning they can be read and replicated.
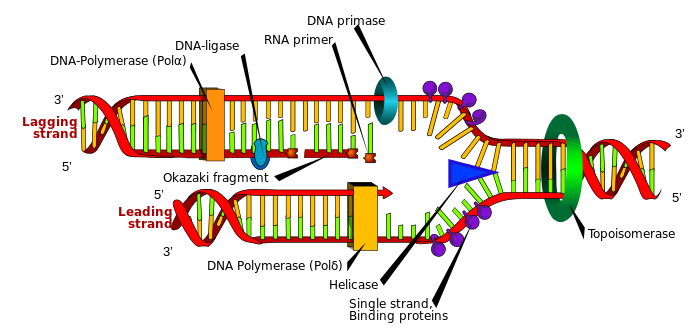
Elongation describes the assembly of new DNA daughter strands from parent strands. The two parent strands can further be classified as leading strand or lagging strand and are distinguished by the continuous or discontinuous direction of replication, respectively. A short fragment of RNA nucleotides acts as a primer, which binds to the parent DNA strand that will be copied. The leading strand receives one primer and the lagging strand receives several. Elongation proceeds with help from enzymes called DNA polymerases, which read parent template strands in a specific direction. Complementary nucleotides are added, and the newly formed daughter strand will grow. The direction in which replication proceeds depends on whether it is the leading or lagging strand. On the leading parent strand, a DNA polymerase will create one continuous strand. Because the lagging parent strand requires several primers, disjointed strands (called Okazaki fragments) will be generated. Other enzymes will fill in the missing nucleotide gaps between the disconnected lagging strand Okazaki fragments.
Finally, termination refers to the end of DNA replication activity. It is signaled by a stop sequence in the DNA, which is recognized by machinery at the replication fork. The end result of DNA replication is that the number of chromosomes are doubled so that the cell can divide into two.
DNA Mutations
DNA replication should result in the creation of two molecules with identical DNA nucleotide sequences. Although DNA polymerases are quite precise during DNA replication, copying mistakes are estimated to occur every 107 DNA nucleotides. Variation from the original DNA sequence is known as a mutation. Briefly, mutations can result in single nucleotide changes as well as the insertion or deletion of nucleotides and repeated sequences. Depending on where they occur, some mutations can be deleterious (harmful). For example, mutations may occur in regions that control cell cycle regulation, which can result in cancer. Many other mutations, however, are not harmful to an organism.
Regardless of their effect, the cell attempts to reduce the frequency of mutations that occur during DNA replication. To accomplish this, there are polymerases with proofreading capacities that can identify and correct mismatched nucleotides. These safeguards reduce the frequency of DNA mutations so that they only occur every 109 nucleotides.
Protein Synthesis
Proteins are strings of amino acids that fold into complex 3-D shapes. There are 20 standard amino acids that can be strung together in different orders in humans, and the result is that proteins can perform an impressive amount of different functions. For instance, muscle fibers are proteins that help facilitate movement. A special class of proteins (immunoglobulins) help protect the organism by detecting disease-causing pathogens in the body. Protein hormones, such as insulin, help regulate physiological activity. Blood hemoglobin is a protein that transports oxygen throughout the body. Enzymes are also proteins, and they are catalysts for biochemical reactions that occur in the cell (e.g., metabolism). Larger-scale protein structures can be visibly seen as physical features of an organism (e.g., hair and nails).
Transcription and Translation
Coding nucleotides in our DNA provide instructions on how to make proteins. Making proteins, also known as protein synthesis, can be broken down into two main steps referred to as transcription and translation. Protein synthesis relies on many molecules in the cell including different types of regulatory proteins and RNAs for each step in the process. Although there are many different types of RNA molecules that have a variety of functions within the cell, we will mainly focus on messenger RNA (mRNA).
A gene is a segment of DNA that codes for RNA, and genes can vary in length from a few hundred to as many as two million base pairs in length. The purpose of transcription is to make an RNA copy of that genetic code (Figure 12). Unlike double-stranded DNA, RNA molecules are single-stranded nucleotide sequences (refer back to Figure 6). Additionally, while DNA contains the nucleotide thymine (T), RNA does not—instead, its fourth nucleotide is uracil (U). Uracil is complementary to (or can pair with) adenine (A), while cytosine (C) and guanine (G) continue to be complementary to each other. For transcription to proceed, a gene must first be turned “on” by the cell. The double-stranded DNA is then separated, and one side of the DNA strand is used as a template where complementary RNA nucleotides are strung together. For example, if a DNA template is TACGGATGC, then the newly constructed mRNA sequence will be AUGCCUACG. Sometimes the end product needed by the cell is that transcribed RNA, but for protein synthesis constructing the RNA (specifically pre-messenger RNA, or pre-mRNA) is just the first step.
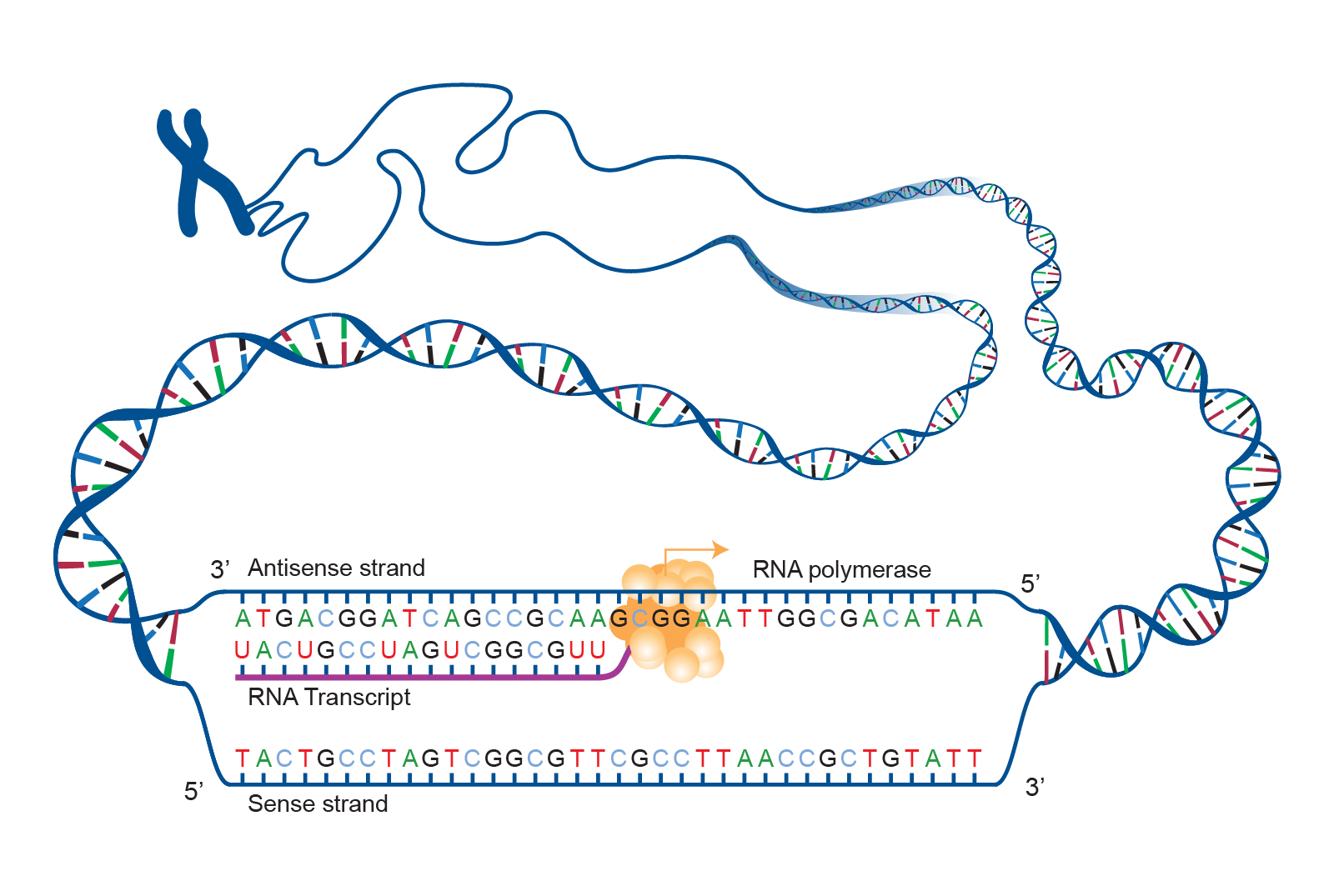
Genes contain segments called introns and exons. Exons are considered “coding” while introns are considered “noncoding”—meaning the information they contain will not be needed to construct proteins. When a gene is first transcribed into pre-mRNA, introns and exons are both included (Figure 13). However, once transcription is finished, introns are removed in a process called splicing. During splicing, a protein/RNA complex attaches itself to the pre-mRNA and removes introns and then connects the remaining exons, thus creating a shorter mature mRNA.
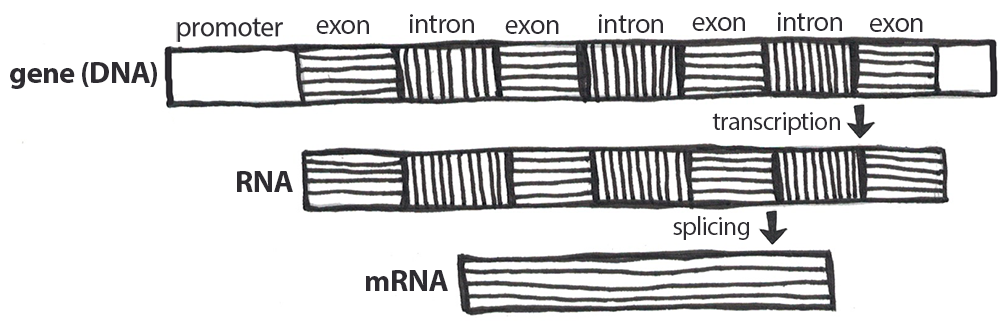
The process by which mRNA is “read” and amino acids chained together to form new proteins is called translation. During translation, mature mRNA is transported outside of the nucleus where it is bound to a ribosome (Figure 14). The nucleotides in the mRNA are read as triplets, which are called codons. Each codon corresponds to an amino acid, and this is the basis for building a protein. Continuing with our example from above, the mRNA sequence AUG-CCU-ACG codes for three amino acids. AUG is a codon for methionine (Met), CCU is proline (Pro), and ACG is threonine (Thr). Therefore, the protein sequence is Met-Pro-Thr. Methionine is the most common “start codon” (AUG) for the initiation of protein translation in eukaryotes. As the ribosome moves along the mRNA, the growing amino acid chain exits the ribosome and folds into a protein (Figure 15). When the ribosome reaches a “stop” codon (UAA, UAG, or UGA), the ribosome stops adding new amino acids, detaches from the mRNA, and the protein is released. Folded proteins can then be used to complete a structural or functional task.
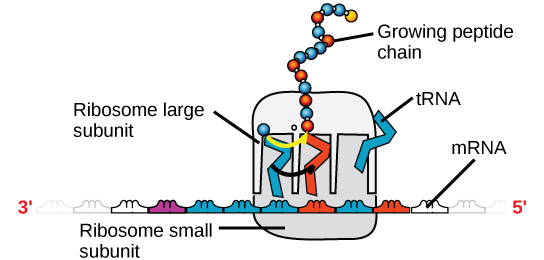
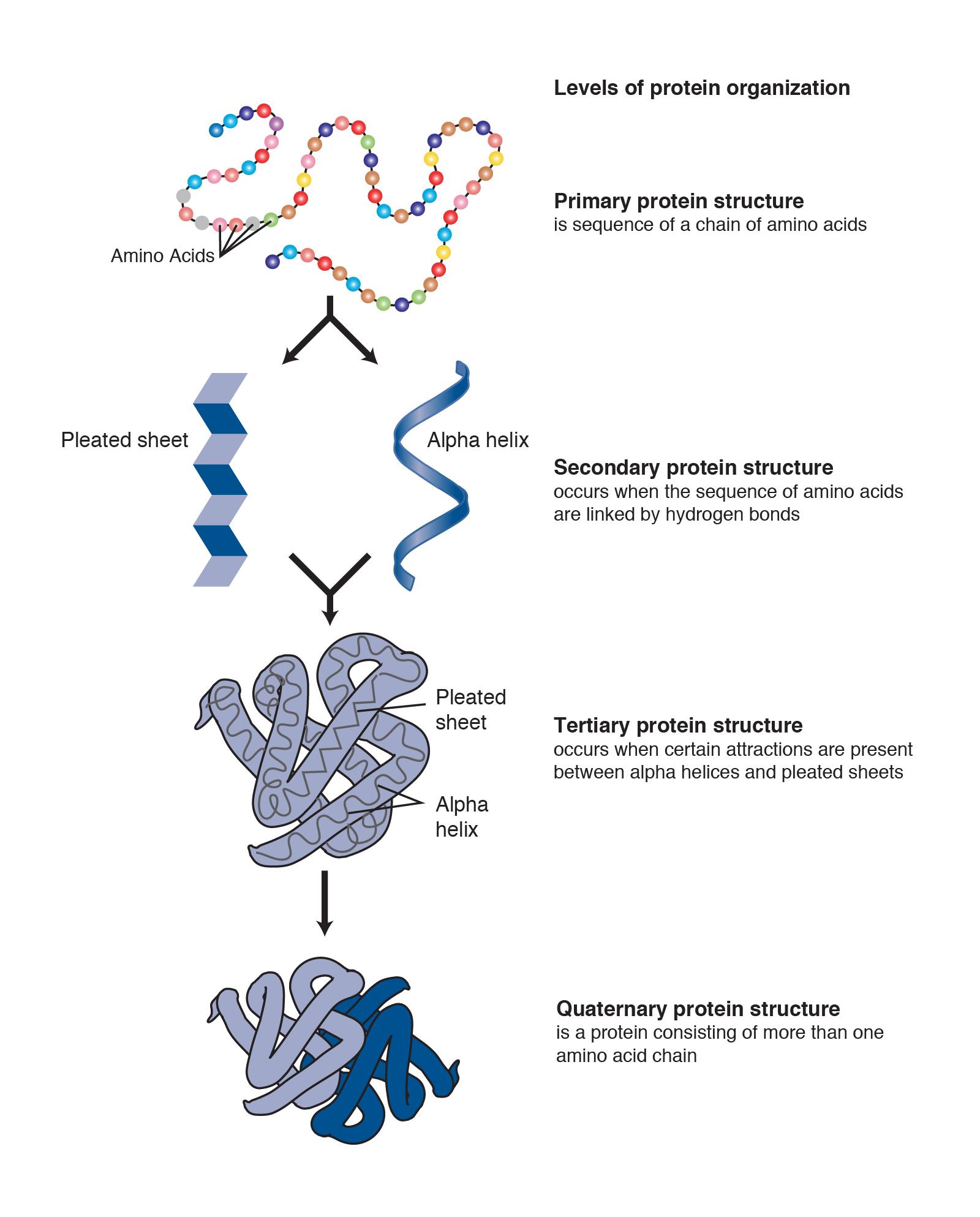
Adaptations
Survival and reproduction at high altitudes (as well as other extreme environments!) present numerous physiological challenges for most humans, but what if there were some humans who were specially adapted to life at high altitudes? These behavioral, acclimatory, and developmental adjustments are all related to the phenotypic plasticity of the individual; however, most adjustments are temporary in nature and they affect a single individual rather than all individuals within a population. But, what if the physiological changes were permanent? What if they affected all members of a population rather than just a single individual? The long-term, micro-evolutionary (i.e., genetic) changes that occur within a population in response to an environmental stressor are referred to as an adaptation. From an evolutionary standpoint, the term “adaptation” refers to a phenotypic trait (i.e., physiological/morphological feature or behavior) that has been acted upon by natural selection processes to increase a species’ ability to survive and reproduce within a specific environment. Within the field of physiology, the term “adaptation” refers to traits that serve to restore homeostasis. The physiology-based interpretation of adaptations presumes that all traits serve a purpose and that all adaptations are beneficial in nature; however, this may be a fallacy, since some traits may be present without clear evidence as to their purpose. As such, during the following discussion of various forms of adaptations in human populations, we will focus our attention to phenotypic traits with an evidence-based purpose.
Adaptation: Altitude
There is genomic research supporting the evolutionary selection of certain phenotypes and their corresponding genotypes within indigenous high-altitude populations across the globe. The following discussion focuses on three high-altitude indigenous populations from Tibet, the Andes, and Ethiopia (Figure 16). Although these populations share many common genetic traits based on relatively similar evolutionary histories influenced by similar environmental stressors, there is support for local genetically based adaptation as well, based on different genes being acted upon by environmental stressors that may be unique to Tibet, the Andes, and Ethiopia (Bigham 2016).
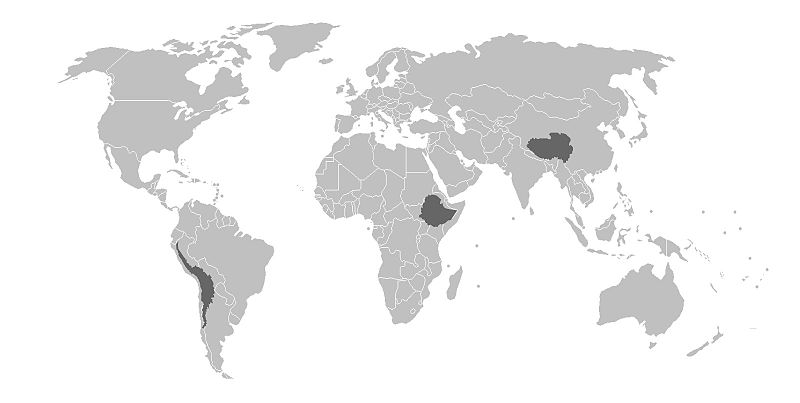
Tibetan populations have resided in the Tibetan Plateau and Himalayan Mountain regions at elevations exceeding 4,000 m.a.s.l. (13,100 f.a.s.l.) for at least the past 7,400 years (Meyer et al. 2017). A gene referred to as EPAS1 is involved in the regulation of red blood cell (and hemoglobin) production as well as catecholamine homeostasis. Catecholamines are hormones (e.g., epinephrine) secreted as part of the sympathetic nervous system’s acute stress (“fight-or-flight”) response. An acute stress response typically includes an increase in: heart rate, blood pressure, and blood glucose levels. Long-term elevation of catecholamines in the body may lead to hypertension (elevated blood pressure), increased blood pH levels, the development of a form of cardiovascular disease leading to narrowing of the arteries (atherosclerosis), and blood clots. In the short term, an acclimatory adjustment leading to the increase of catecholamines and hemoglobin production by the hypoxia (low-oxygen level)-induced activation of the EPAS1 gene may assist individuals from lower altitudes as they ascend to significantly higher altitudes; however, such increases may not be maintained for long before they cause damage to the body. For indigenous high-altitude populations of Tibet, a mutation in the EPAS1 gene inhibits increased red blood cell production and assists with catecholamine regulation. The red blood cell count of high-altitude Tibetans with the EPAS1 point mutation is about the same as for individuals residing at sea level.
Interestingly, individuals in populations from the high-altitude Andean Altiplano of Peru and Bolivia, such as the Quechua and Aymara, lack the EPAS1 point mutation, so their red blood cell counts are relatively elevated compared with the Tibetan populations. In addition, populations from the Andean Altiplano also have an increased arterial oxygen saturation and low, hypoxia-induced breathing pattern (minor hyperventilation) as compared to their Tibetan counterparts (Bigham 2016). Populations indigenous to the Semien Plateau of Ethiopia, such as the Oromo and Amhara, share a similar but not identical EPAS1 point mutation with the Tibetan population (Bigham 2016). This suggests that the EPAS1 mutations occurred independently from each other; however, their effects are still similar in that they permit the Tibetan and Ethiopian populations to survive at high altitudes. Not all adaptations are related to life in high-altitude environments, however. In the following sections, we will address more general examples of adaptation in human populations: variations in skin color and differences in body build.
Adaptation: Skin Tone
When you think about your own skin tone and compare it to members of your family, do you all possess exactly the same shade? Are some members of your family darker than others? What about your friends? Your classmates? Skin tone occurs along a continuum, which is a reflection of the complex evolutionary history of our species. The expression of skin tone is regulated primarily by both melanin and hemoglobin. Melanin is a dark brown-black pigment that is produced by the oxidation of certain amino acids (e.g., tyrosine, cysteine, phenylalanine) in the melanocytes, which are specialized cells located in the base layer (stratum basale) of the skin’s epidermis as well as several other areas within the body (Figure 17). Within the melanocytes, melanin is produced in the special organelle called a melanosome. Melanosomes serve as sites for the synthesis, storage, and transportation of melanin. Melanosomes transport the melanin particles through cellular projections to epidermal skin cells (keratinocytes) as well as to the base of the growing hair root (root sheath portion). In the eye, however, melanin particles produced by the melanosomes remain present within the iris (iridial melanocytes) and are not transported beyond their origin location. The two main forms of melanin related to skin, hair, and eye color are eumelanin and pheomelanin. All humans contain both eumelanin and pheomelanin within their bodies; however, the relative expression of these two forms of melanin determines an individual’s overall coloring. Eumelanin is a brown-to-black colored melanin particle and pheomelanin is more pink-to-red. Individuals with darker skin or hair color have a greater expression of eumelanin than those with lighter-colored skin and blonde or red hair.
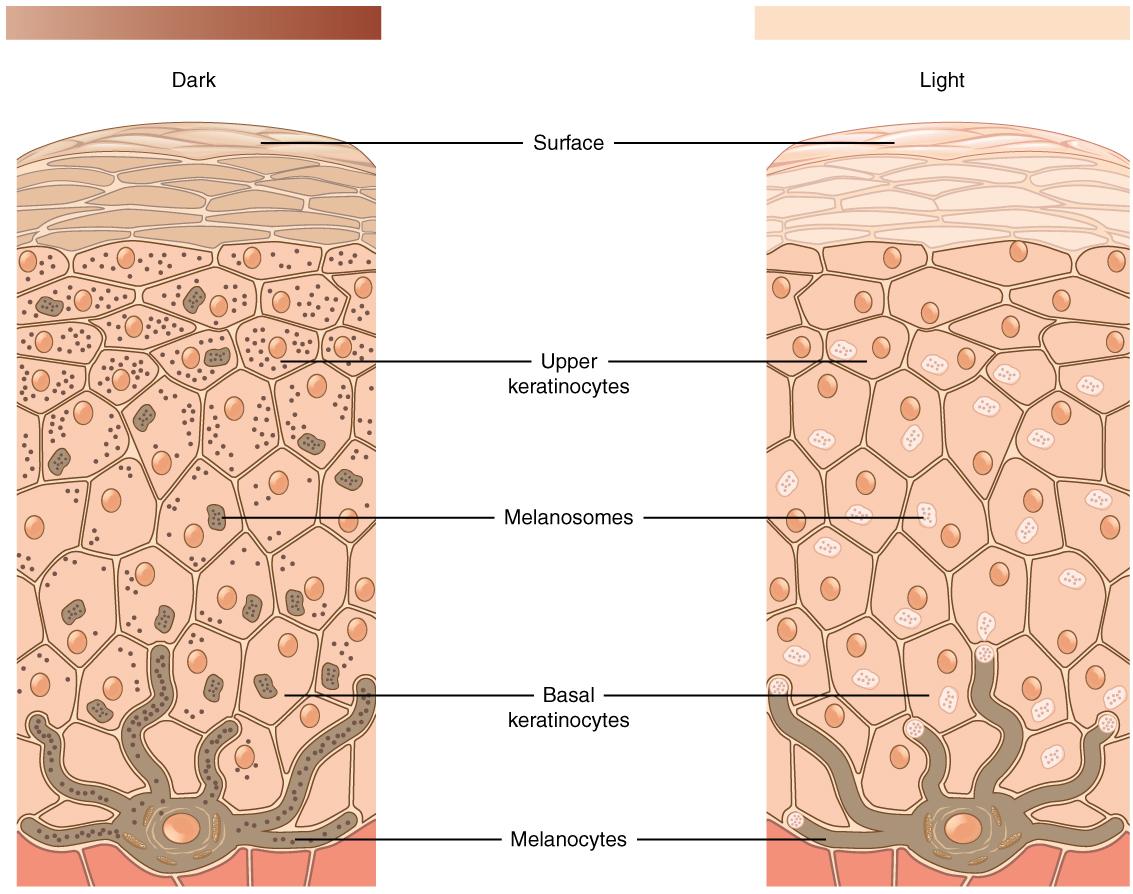
Skin Tone Genetic Regulation
The melanocortin 1 receptor (MC1R) gene acts to control which types of melanin (eumelanin or pheomelanin) are produced by melanocytes. The MC1R receptor is located on the surface of the melanocyte cells (Quillen et al. 2018). Activation of the MC1R receptors may occur through exposure to specific environmental stimuli or due to underlying genetic processes. Inactive or blocked MC1R receptors results in melanocytes producing pheomelanin. If the MC1R gene receptors are activated, then the melanocytes will produce eumelanin. Thus, individuals with activated MC1R receptors tend to have darker pigmented skin and hair than individuals with inactive or blocked receptors.
The alleles of another gene, the major facilitator, superfamily domain-containing protein 12 (MFSD12) gene, affect the expression of melanocytes in a different way than the MC1R gene. Instead of affecting the activation of melanocyte receptors, the MFSD12 alleles indirectly affect the membranes of melanocyte lysosomes (Quillen et al. 2018). The melanocyte’s lysosomes are organelles containing digestive enzymes, which ultimately correlate to varying degrees of pigmentation in humans. Variations in the membranes of the melanocyte lysosomes ultimately correlate to differing degrees of pigmentation in humans.
Ancestral MFSD12 allele variants are present in European and East Asian populations and are associated with lighter pigmentation of the skin (Crawford et al. 2017; Quillen et al. 2018). In addition, this ancestral variant is also associated with Tanzanian, San, and Ethiopian populations of Afro-Asiatic ancestry (Crawford et al. 2017; Quillen et al. 2018). In contrast, the more-derived (i.e. more recent) allele variants that are linked to darker skin tones are more commonly present in East African populations, particularly those of Nilo-Saharan descent (Crawford et al. 2017; Quillen et al. 2018). The notion that ancestral alleles of MFSD12 are associated with lighter skin pigmentation is in opposition to the commonly accepted idea that our pigmentation was likely darker throughout early human evolution (Crawford et al. 2017; Quillen et al. 2018). Due to the complexity of the human genome, MFSD12 and MC1R are but two examples of alleles affecting human skin tone. Furthermore, there is genetic evidence suggesting that certain genomic variants associated with both darker and lighter skin color have been subject to directional selection processes for as long as 600,000 years, which far exceeds the evolutionary span of Homo sapiens sapiens (Crawford et al. 2017; Quillen et al. 2018). So, evolutionary processes may lead to skin becoming more darkly pigmented as well as more lightly pigmented.
Although all humans have approximately the same number of melanocytes within the epidermis, the production of melanin by these melanocytes varies. There are two forms of melanogenesis (the process through which melanocytes generate melanin): basal and activated. As discussed previously, the expression of eumelanin and pheomelanin by the melanocytes is genetically regulated through the expression of specific receptors (e.g., MC1R) or other melanocyte components (e.g., MFSD12). Basal melanogenesis is dependent upon an individual’s inherent genetic composition and is not influenced by external factors. Activated melanogenesis occurs in response to ultraviolet radiation (UV) exposure, specifically UV-B (short UV wave) exposure. Increased melanogenesis in response to UV-B exposure serves to provide protection to the skin’s innermost layer called the hypodermis, which lies below the epidermis and dermis (Figure 14.11). Melanin in the skin, specifically eumelanin, effectively absorbs UV-B radiation from light meaning that it will not reach the hypodermal layer. This effect is often more apparent during periods of the year when individuals tend to be outside more and the weather is warmer, which leads to those individuals donning fewer protective garments. The exposure of skin to sunlight is, of course, culturally mediated with some cultures encouraging the covering of skin at all times.
As previously noted, hemoglobin is an iron-rich protein that binds with oxygen in the bloodstream. For individuals with lighter-colored skin, blood vessels near the surface of the skin and the hemoglobin contained within those vessels is more apparent than in individuals with darker skin. The visible presence of hemoglobin coupled with the pink-to-red tone of the pheomelanin leads to lighter-skinned individuals having a pale pink skin tone. Individuals with lighter skin more readily absorb UV radiation as their basal melanin expression is directed more toward the production of pheomelanin than eumelanin. But, why are there so many variations in skin tone in humans? To answer this question, we now turn toward an exploration of an evolutionary-based adaptation of skin tone as a function of the environment.
Evolutionary Basis for Skin Tone Variation
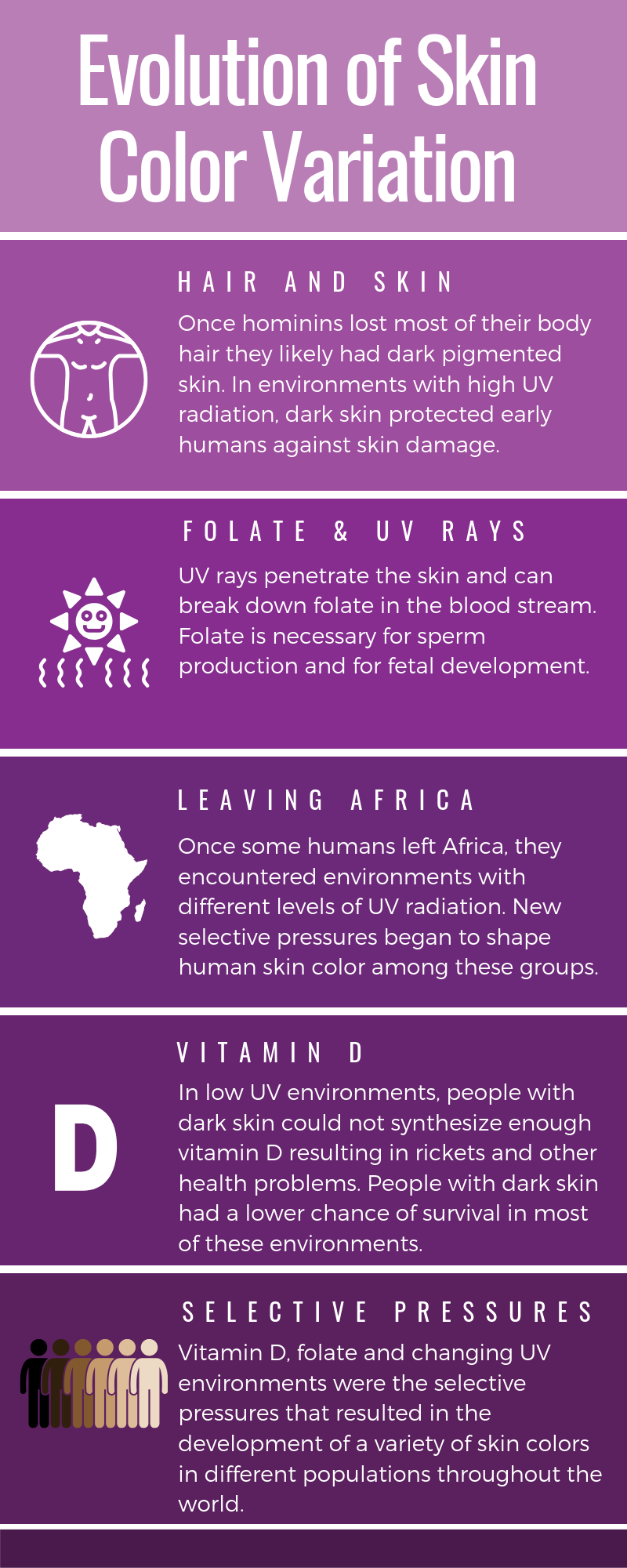
Skin cancer is a significant concern for many individuals with light skin tone as the cumulative exposure of the epidermis and underlying skin tissues to UV radiation may lead to the development of abnormal cells within those tissues leading to malignancies. Although darker-skinned individuals are at risk for skin cancer as well, they are less likely to develop it due to increased levels of melanin, specifically eumelanin, in their skin. Even though skin cancer is a serious health concern for some individuals, most skin cancers occur in the post-reproductive years; therefore, it is improbable that evolutionary forces favoring varying melanin expression levels are related to a selective pressure to avoid such cancers. Furthermore, if avoiding skin cancer were the primary factor driving the evolution of various skin tones, then it reasons that everyone would have the most significant expression of eumelanin possible. So, why do we have different skin tones (Figure 18)? The term cline refers to the continuum or spectrum of gradations (i.e., levels or degrees) from one extreme to another. With respect to skin tone, the various tonal shades occur clinally, with darker skin being more prevalent near the equator and gradually decreasing in tone (i.e., decreased melanin production) in more distant latitudes. For individuals who are indigenous to equatorial regions, the increased levels of melanin within their skin provides them with a measure of protection against both sunburn and sunstroke as the melanin is more reflective of UV radiation than hemoglobin. In cases of severe sunburn, eccrine glands are affected, resulting in an individual’s ability to sweat being compromised. As sweat is the body’s most effective means of reducing its core temperature to maintain homeostasis, damage to the eccrine glands may lead to numerous physiological issues related to heat that may ultimately result in death.
Even though avoiding severe sunburn and sunstroke is of great importance to individuals within equatorial regions, this is likely not the primary factor driving the evolutionary selection of darker skin within these regions. It has been proposed that the destruction of folic acid, which is a form of B-complex vitamin, by UV radiation may have led to the selection of darker skin in equatorial regions. For pregnant women, low levels of folic acid within the body during gestation may lead to defects in the formation of the brain and spinal cord of the fetus. This condition, which is referred to as spina bifida, often significantly reduces the infant’s chances of survival without medical intervention. In men, low levels of folic acid within the body lead to an inhibition in the production of sperm. Thus, in geographic regions with high UV radiation levels (i.e., equatorial regions), there appears to be an evolutionarily driven correlation between darker skin and the maintenance of fertility.
If darker skin tone is potentially correlated to more successful reproduction, then why do lighter shades of skin exist? One hypothesis is that there is a relationship between lighter skin tone and vitamin D synthesis within the body. When skin is exposed to the UV-B radiation waves in sunlight, a series of chemical reactions occur within the epidermis leading to the production of vitamin D3. Before the body can use vitamin D3, it must travel to the liver and then to the kidneys where it is converted into different forms of bioactive molecules. Ultimately, it is converted into the bioactive molecule calcitriol. Within the human body there are numerous cell types with binding receptors for calcitriol, so it is capable of adhering to the DNA of those cells. Calcitriol serves as a regulator in cellular-replication processes within the body, including those for pancreatic, breast, colon, and kidney cells. Insufficient calcitriol is associated with an increased risk of: some forms of cancer (colon, prostate, etc.), autoimmune diseases (multiple sclerosis, lupus, type I diabetes, etc.), cardiovascular diseases, and infections (e.g., tuberculosis, influenza). Deficiencies in calcitriol production and absorption within the human body may be linked to underlying genetic factors, such as a mutation in the vitamin D receptors present in some of the body’s cells. Alternatively, it may be linked to inadequate exposure to the UV-B rays necessary to stimulate calcitriol production or to a nutritional deficiency in vitamin D-rich foods. Regardless of the cause of the deficiency, individuals with a calcitriol (vitamin D3) deficiency may also be at risk for the development of certain skeletal abnormalities in addition to the previously mentioned health issues.
Vitamin D is required for the absorption of certain nutrients, such as calcium and phosphorus, in the small intestine. These nutrients are among those that are critical for the proper growth and maintenance of bone tissue within the body. In the absence of adequate minerals, particularly calcium, bone structure and strength will be compromised leading to the development of rickets during the growth phase. Rickets is a disease affecting children during their growth phase and is characterized by inadequately calcified bones that are softer and more flexible than normal. Individuals with rickets will develop a true bowing of their femora, which may affect their mobility. In addition, deformation of pelvic bones in women may occur as a result of rickets leading to complications with reproduction. In adults, a deficiency in vitamin D will often result in osteomalacia, which is a general softening of the bones due to inadequate mineralization. This softening is the result of impaired bone metabolism that is primarily linked to insufficient levels of bioavailable vitamin D, calcium, and phosphate. In addition, it may be linked to inadequate absorption of calcium in the bloodstream. As noted, a variety of maladies may occur due to the inadequate production or absorption of vitamin D, as well as due to the destruction of folate within the human body; so, from an evolutionary perspective, natural selection should favor a skin tone that is best suited to a given environment.
In general, the trend related to lighter skin pigmentation further from the equator follows a principle called Gloger’s Rule This rule states that within the same species of mammals the more heavily pigmented individuals tend to originate near the equator while lighter-pigmented members of the species will be found in regions further from the equator. Gloger’s Rule applies latitudinally; however, it does not appear to hold for certain human populations near the poles. Specifically, the Inuit people (Figure 14.14), who are indigenous to regions near the North Pole and currently reside in portions of Canada, Greenland, and Alaska. The Inuit have a darker skin tone that would not be anticipated under the provisions of Gloger’s Rule. The high reflectivity of light off of snow and ice, which is common in polar regions, necessitates the darker skin tone of these individuals to prevent folic acid degradation just as it does for individuals within equatorial regions. The consumption of vitamin D–rich foods, such as raw fish, permits the Inuit to reside at high latitudes with darker skin tone while preventing rickets.
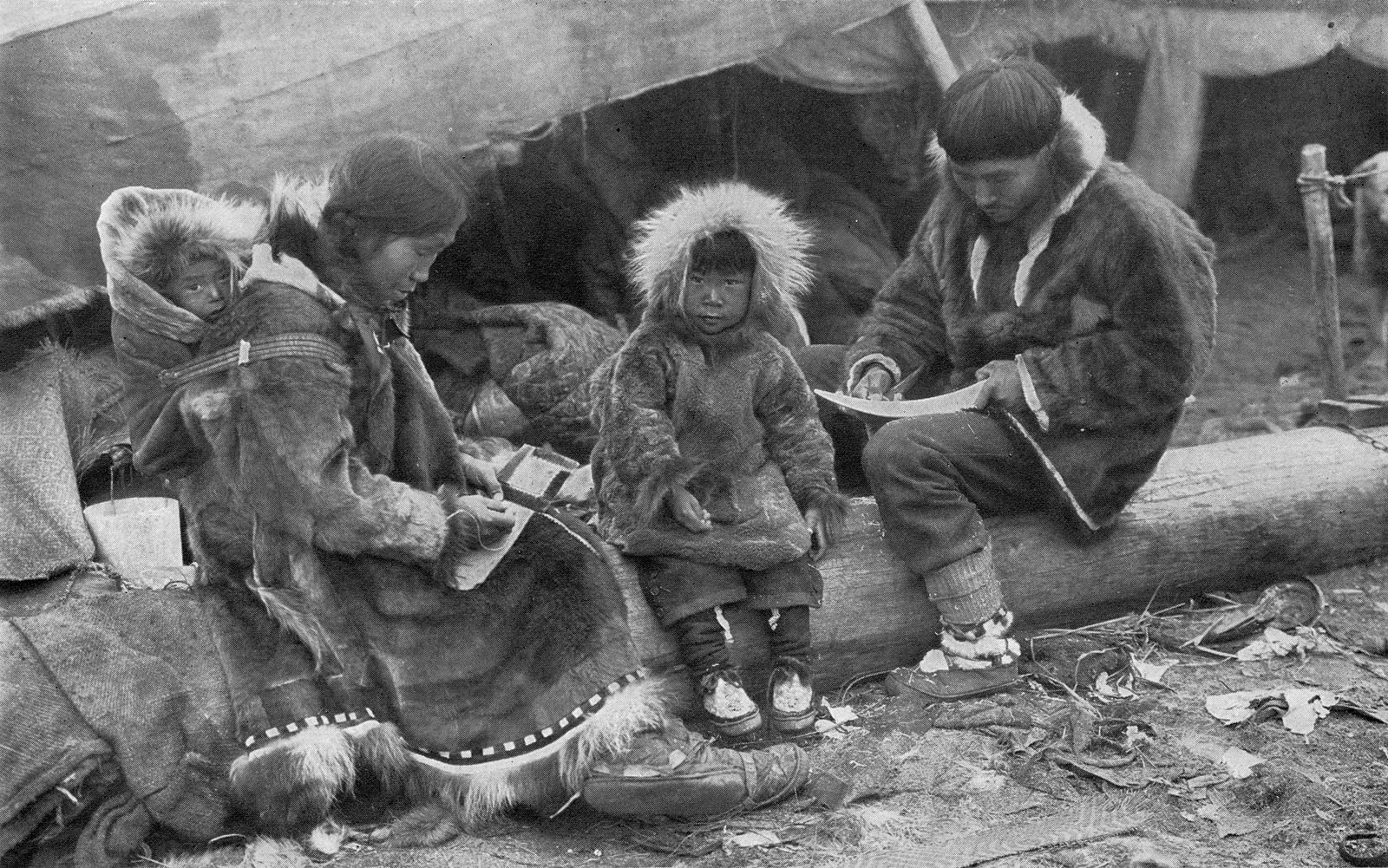
Genome studies have identified a number of genes (TYR, OCA2/HERC2, TYRP1, SLC45A2, HPS6i, etc.) related to the expression of melanin and pigmentation presentation in humans. Compared to the exceptionally large number of genes within the human genome, those regulating the expression of melanin are relatively few and appear on distinct loci. The genes at these loci are generally pleiotropic in nature, so there is a relatively predictable patterning in skin, hair, and eye color combinations. For example, some populations that are indigenous to higher latitude regions tend to have lighter skin, hair, and eye color than their counterparts from equatorial regions. Still, since the genes affecting skin, hair, and eye color are actually independent, it is possible that variations may produce many phenotypic combinations. Turning again to our example of individuals indigenous to higher latitudes, it is theoretically possible to encounter an individual with dark hair, light-toned skin, and blue eyes within this region due to the variability of phenotypic combinations.
Adaptation: Shape and Size Variations
In addition to natural selection playing a role in the determination of melanin expression related to skin tone, it plays a significant role in the determination of the shape and size of the human body. The most significant thermodynamic mechanism of heat loss from the body is radiation. At temperatures below 20℃ (68℉), the human body loses around 65% of its heat to radiative processes; however, the efficiency of radiation as a means of heat reduction is correlated to the overall body shape and size of the individual. There is a direct correlation between the ratio of an object’s surface area to mass and the amount of heat that may be lost through radiation. For example, two metal objects of identical composition and mass are heated to the same temperature. One object is a cube and the other is a sphere. Which object will cool the fastest? Geometrically, a sphere has the smallest surface area per unit mass of any three-dimensional object, so the sphere will cool more slowly than the cube. In other words, the smaller the ratio of the surface area to mass an object has, the more it will retain heat. This, in general, holds true for humans, as well.
In regions where temperatures are consistently cold, the body shape and size of the individuals who are indigenous to the area tend to be more compact. These individuals have a relatively higher body mass to surface area (i.e., skin) than their counterparts from equatorial regions where the average temperatures are considerably warmer. Individuals from hot climates, such as the Fulani (Figure 14.15a) of West Africa, have limbs that are considerably longer than those of individuals from cold climates, such as the Inuit of Greenland (Figure 19). Evolutionarily, the longer limbs of individuals from equatorial regions (e.g., the Fulani) provide a greater surface area (i.e., lower body mass to surface area ratio) for the dissipation of heat through radiative processes. In contrast, the relatively short limbs of Arctic-dwelling people, such as the Inuit, allows for the retention of heat as there is a decreased surface area through which heat may radiate away from the body.
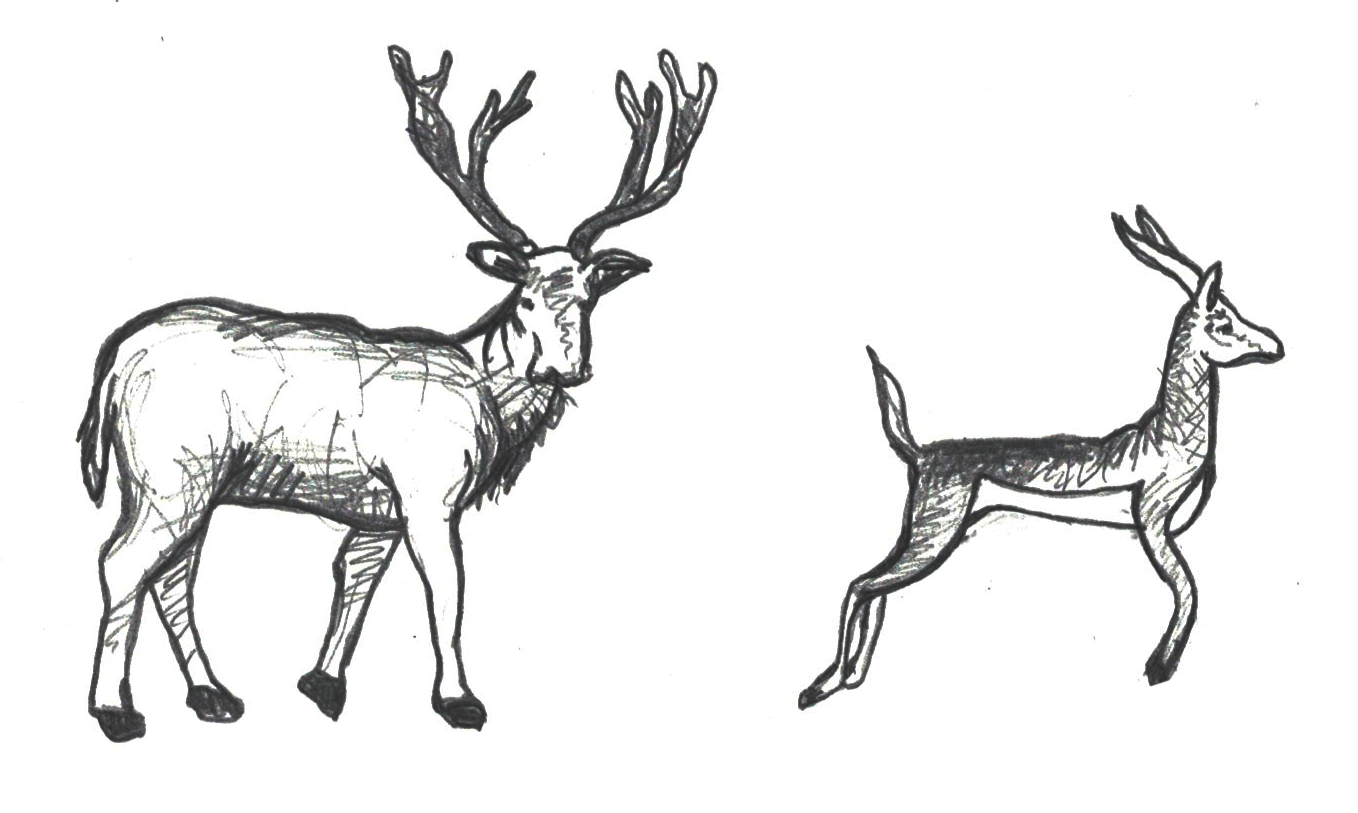
As described above, there are certain trends related to the general shape and size of human bodies in relation to the thermal conditions. To better describe these trends, we turn to a couple of general principles that are applicable to a variety of species beyond humans. Bergmann’s Rule predicts that as average environmental temperature decreases, populations are expected to exhibit an increase in weight and a decrease in surface area (Figure 20). Also, within the same species of homeothermic animals, the relative length of projecting body parts (e.g., nose, ears, and limbs) increases in relation to the average environmental temperature (Figure 21). This principle, referred to as Allen’s Rule, notes that longer, thinner limbs are advantageous for the radiation of excess heat in hot environments and shorter, stockier limbs assist with the preservation of body heat in cold climates. A measure of the crural index (crural index=tibia length [divided by] femur length) of individuals from various human populations provides support for Allen’s Rule since this value is lower in individuals from colder climates than it is for those from hot climates. The crural indices for human populations varies directly with temperature.
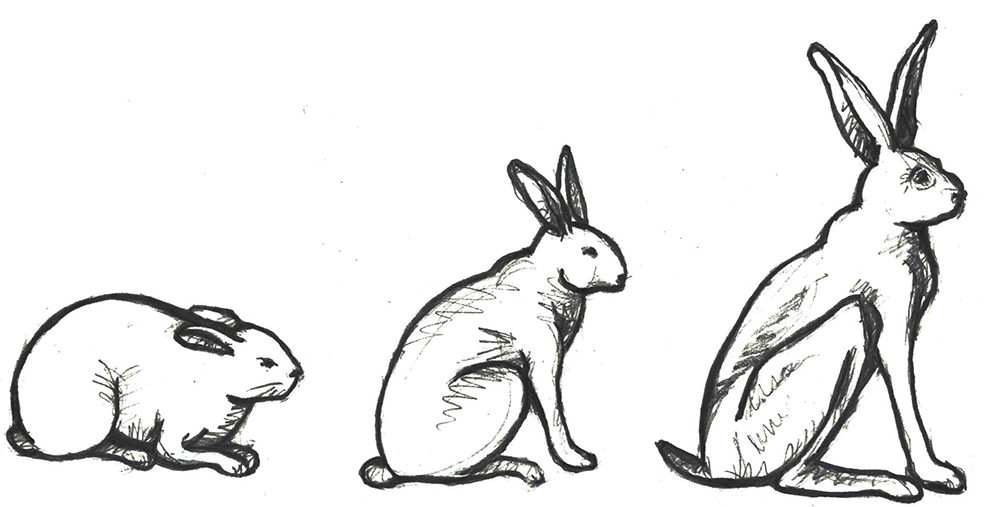
Adaptation: Infectious Disease
Throughout our evolutionary journey, humans have been exposed to numerous infectious diseases. In the following section, we will explore some of the evolutionary-based adaptations that have occurred in certain populations in response to the stressors presented by select infectious diseases. One of the primary examples of natural selection processes acting on the human genome in response to the presence of an infectious disease is the case of the relationship between the sickle-cell anemia trait and malaria.
Malaria is a zoonotic disease, a type of infectious disease naturally transmitted between animals and humans, caused by the spread of the parasitic protozoa from the genus Plasmodium. These unicellular, eukaryotic protozoa are transmitted through the bite of a female Anopheles mosquito. During the bite process, the protozoan parasites that are present within an infected mosquito’s saliva will enter the bloodstream of the individual where they will be transported to the liver. Within the liver, the parasites multiply and will eventually be released into the bloodstream where they will infect erythrocytes (red blood cells). Once inside the erythrocytes, the parasites will reproduce until they exceed the cell’s storage capacity, causing it to burst and release the parasites into the bloodstream once again. This replication cycle will continue as long as there are viable erythrocytes within the host to infect.
General complications from malaria infections include: enlargement of the spleen (due to destruction of infected erythrocytes), lower number of thrombocytes (also called platelets, required for coagulation/clotting of blood), high levels of bilirubin (a byproduct of hemoglobin breakdown in the liver) in the blood, jaundice (yellowing of the skin and eyes due to increased blood bilirubin levels), fever, vomiting, retinal (eye) damage, and convulsions (seizures). According to the World Health Organization, in 2022 there were 608,000 deaths from malaria globally, with the highest percentage of those deaths occurring in Africa (95%) (World Health Organization 2023). In sub-Saharan Africa, where incidence of malaria is the highest in the world, millions of pregnancies are affected by malaria, resulting in thousands infant deaths. Pregnant women who become infected during the gestational process are more likely to have low-birthweight infants due to prematurity or growth restriction inside the uterus (Hartman et al. 2013). After birth, infants born to malaria-infected mothers are more likely to develop infantile anemia (low red blood cell counts), a malaria infection that is not related to the maternal malarial infection, and they are more likely to die than infants born to non-malaria-infected mothers.
For children and adolescents whose brains are still developing, there is a risk of cognitive (intellectual) impairment associated with some forms of malaria infections (Fernando et al. 2010). Given the relatively high rates of morbidity (disease) and mortality (number of deaths) associated with malaria, it leads to reason that this disease may have served as a selective pressure during human evolution. Support for natural selection related to malaria resistance is related to genetic mutations associated with sickle cell, thalassemia, glucose-6-phosphate dehydrogenase (G6PD) deficiency, and the absence of certain antigens (molecules capable of inducing an immune response from the host) on erythrocytes. For the purposes of this text, we will focus our discussion on the relationship between sickle cell disease and malaria.
Sickle cell disease is a group of genetically inherited blood disorders characterized by an abnormality in the shape of the hemoglobin within erythrocytes. It is important to note that there are multiple variants of hemoglobin. Each of these variants of hemoglobin may result in various conditions within the body; however, for the following explanation we will focus solely on variants A and S.
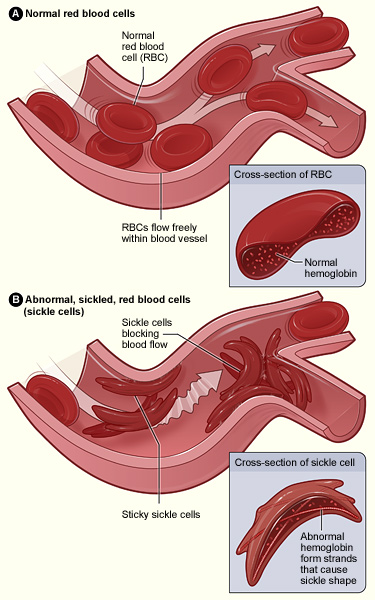
Individuals who inherit a mutated gene (hemoglobin with a sickled erythrocyte variety, HbS) on chromosome 11 from both parents will develop sickle cell anemia, which is the most severe form of the sickle cell disease family (Figure 22). The genotype of an individual with sickle cell anemia is HbSS; whereas, an individual without sickle cell alleles has a genotype of HbAA representing two normal adult hemoglobin type A variants. Manifestations of sickle cell anemia (HbSS) range from mild to severe with some of the more common symptoms being: anemia, blood clots, organ failure, chest pain, fever, and low blood oxygen levels. In high-income countries with advanced medical care, the median life expectancy of an HbSS individual is around 60 years; however, in low-income countries where advanced medical care is scarce, as many as 90% of children with sickle cell disease perish before the age of five (Longo et al. 2017).
Considering that advanced medical care was not available during much of human evolutionary history, it stands to reason that the majority of individuals with the HbSS genotype died before the age of reproduction. If that is the case though, why do we still have the HbS variant present in modern populations? As covered earlier in this textbook, the genotype of an individual is composed of genes from both biological parents. In the case of an individual with an HbSS genotype, the sickle cell allele (HbS) was inherited from each of the parents. For individuals with the heterozygous genotype of HbSA, they have inherited both a sickle cell allele (HbS) and a normal hemoglobin allele (HbA). Heterozygous (HbSA) individuals who reside in regions where malaria is endemic may have a selective advantage. They will experience a sickling of some, but not all, of their erythrocytes. Unlike an individual with the HbSS genotype, someone with HbSA may experience some of the symptoms listed above; however, they are generally less severe.
As noted earlier, the mechanism through which Plasmodium protozoan parasites replicate involves human erythrocyte cells. Due to their sickled shape, as well as the presence of an abnormally shaped protein within the cell, the parasites are unable to replicate effectively in the erythrocyte cells coded for by the HbS allele (Cyrklaff et al. 2011). An individual who has an HbSA genotype and an active malaria infection will become ill with the disease to a lesser extent than someone with an HbAA genotype. Although normal erythrocytes (regulated by the HbA allele) allow for the replication of the parasite, the parasites will not be able to replicate in HbS erythrocytes of the heterozygote. So, individuals with the HbSA genotype are more likely to survive a malaria infection than an individual who is HbAA. Although individuals with the HbSA genotype may endure some physiological complications related to the sickling of some of their erythrocytes, their morbidity and mortality rates are lower than they are for HbSS members of the population. The majority of individuals who are heterozygous or homozygous for the HbS trait have ancestors who originated in places where malaria was endemic, such as sub-Saharan Africa, India, Saudi Arabia, regions in South and Central America, the Caribbean, and the Mediterranean (Turkey, Greece, and Italy)
With respect to the history of these regions, during the early phases of settlement horticulture was the primary method of crop cultivation. Typically performed on a small scale, horticulture is based on manual labor and relatively simple hand tools rather than the use of draft animals or irrigation technologies. Common in horticulture is swidden, or the cutting and burning of plants in woodland and grassland regions. The swidden is the prepared field that results following a slash-and-burn episode. This practice fundamentally alters the soil chemistry, removes plants that provide shade, and increases the areas where water may pool. This anthropogenically altered landscape provides the perfect breeding ground for the Anopheles mosquito, as it prefers warm, stagnant pools of water (Figure 23).
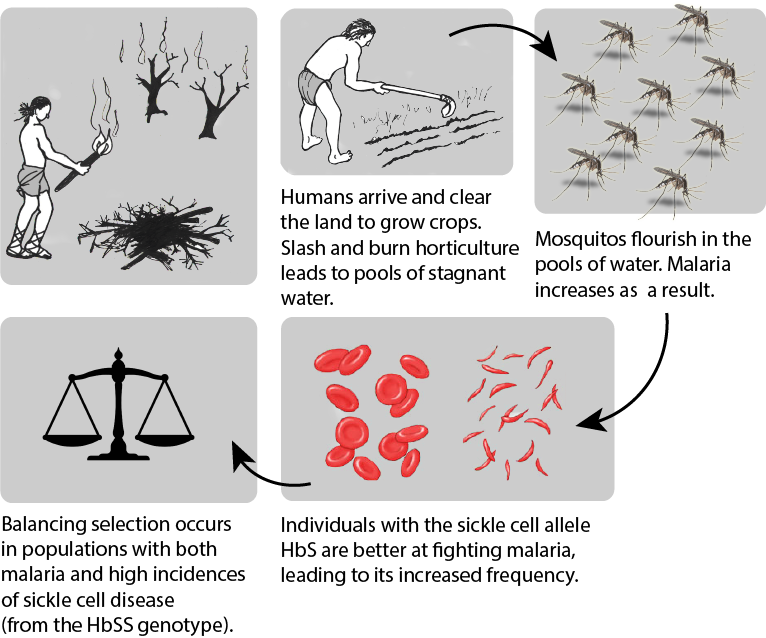
Although swidden agriculture was historically practiced across the globe, it became most problematic in the regions where the Anopheles mosquito is endemic. These areas have the highest incidence rates of malaria infection. Over time, the presence of the Anopheles mosquito and the Plasmodium parasite that it transmitted acted as a selective pressure, particularly in regions where swidden agricultural practices were common, toward the selection of individuals with some modicum of resistance against the infection. In these regions, HbSS and HbSA individuals would have been more likely to survive and reproduce successfully. Although individuals and populations are far more mobile now than they have been throughout much of history, there are still regions where we can see higher rates of malaria infection as well as greater numbers of individuals with the HbS erythrocyte variant. The relationship between malaria and the selective pressure for the HbS variant is one of the most prominent examples of natural selection in the human species within recent evolutionary history.
Adaptation: Lactase Persistence
With the case of sickled erythrocytes and their resistance to infection by malaria parasites, there is strong support for a cause-and-effect-style relationship linked to natural selection. Although somewhat less apparent, there is a correlation between lactase persistence and environmental challenges. Lactase-phlorizin hydrolase (LPH) is an enzyme that is primarily produced in the small intestine and permits the proper digestion of lactose, a disaccharide (composed of two simple sugars: glucose and galactose) found in the milk of mammals. Most humans will experience a decrease in the expression of LPH following weaning, leading to an inability to properly digest lactose. Generally, LPH production decreases between the ages of two and five and is completely absent by the age of nine (Dzialanski et al. 2016). For these individuals, the ingestion of lactose may lead to a wide variety of gastrointestinal ailments including abdominal bloating, increased gas, and diarrhea. Although the bloating and gas are unpleasant, the diarrhea caused by a failure to properly digest lactose can be life-threatening if severe enough due to the dehydration it can cause. Some humans, however, are able to produce LPH far beyond the weaning period.
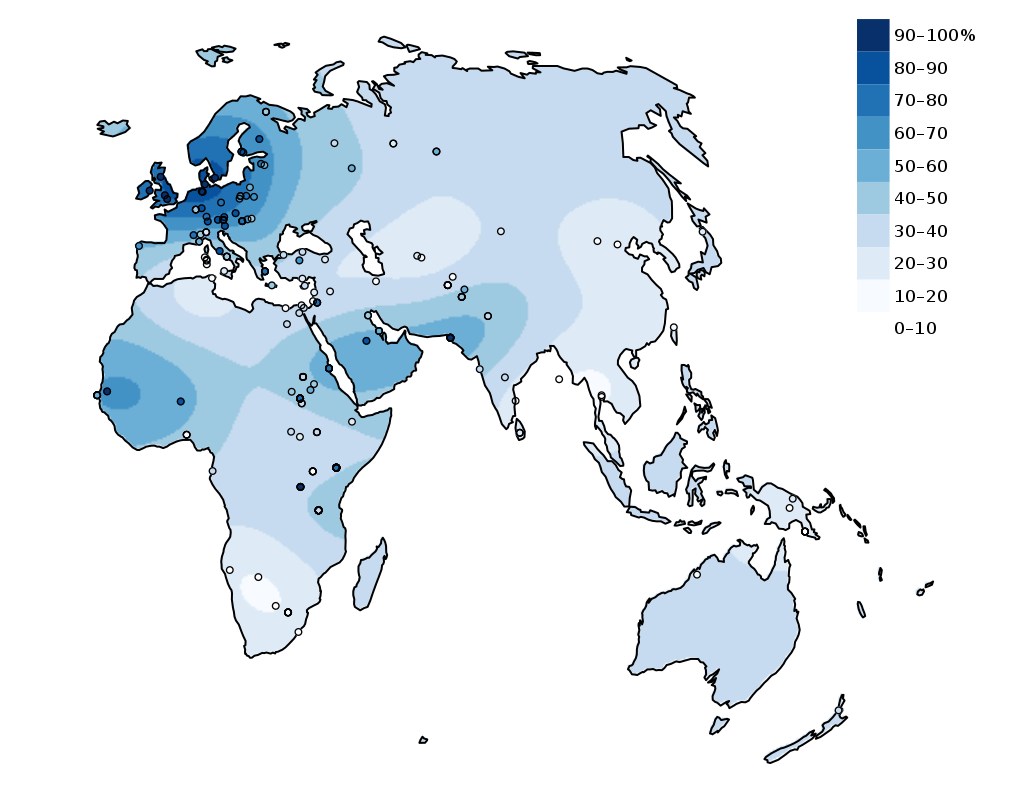
Individuals who continue to produce LPH have what is referred to as the lactase persistence trait. The lactase persistence trait is encoded for a gene called LCT, which is located on human chromosome 2. From an evolutionary and historical perspective, this trait is most commonly linked to cultures that have practiced cattle domestication (Figure 24). For individuals in those cultures, the continued expression of LPH may have provided a selective advantage. During periods of environmental stress, such as a drought, if an individual is capable of successfully digesting cow’s milk, they have a higher chance of survival than someone who suffers from diarrhea-linked dehydration due to a lack of LPH. Per Tishkoff et al. , the “frequency of lactase persistence is high in northern European populations (more than 90% in Swedes and Danes), decreases in frequency across southern Europe and the Middle East (less than 50% in Spanish, French, and pastoralist Arab populations), and is low in non-pastoralist Asian and African populations (less than 1% in Chinese, less than 5% to 20% in West African agriculturalists)” (2007: 248). Although the frequency of the lactase persistence trait is relatively low among African agriculturalists, it is high among pastoralist populations that are traditionally associated with cattle domestication, such as the Tutsi and Fulani, who have frequencies of 90% and 50%, respectively (Tishkoff et al. 2007).
It appears that the emergence of the lactase persistence mutation in European vs. non-European populations, specifically those in East Africa (e.g., Tutsi and Fulani), is a case of convergent evolution. With convergent evolution events, a similar mutation may occur in species of different lineages through independent evolutionary processes. Based on our current understanding of the genetic mutation pathways for the lactase persistence trait in European and African populations, these mutations are not representative of a shared lineage. In other words, just because a person of European origin and a person of African origin can each digest milk due to the presence of the lactase-persistence trait in their genotypes, it does not mean that these two individuals inherited it due to shared common ancestry.
Human Variation: Our Story Continues
From the time that the first of our species left Africa, we have had to adjust and adapt to numerous environmental challenges. The remarkable ability of human beings to maintain homeostasis through a combination of both nongenetic (adjustments) and genetic (adaptations) means has allowed us to occupy a remarkable variety of environments from high-altitude mountainous regions to the tropics near the equator. From adding piquant, pungent spices to our foods as a means of inhibiting food-borne illnesses due to bacterial growth to donning garments specially suited to local climates, behavioral adjustments have provided us with a nongenetic means of coping with obstacles to our health and well-being. Acclimatory adjustments, such as sweating when we are warm in an attempt to regulate our body temperature or experiencing increased breathing rates as a means of increasing blood oxygen levels in regions where the partial pressure of oxygen is low, have been instrumental in our survival with respect to thermal and altitudinal environmental challenges. For some individuals, developmental adjustments that were acquired during their development and growth phases (e.g., increased heart and lung capacities for individuals from high-altitude regions) provide them with a form of physiological advantage not possible for someone who ventures to such an environmentally challenging region as an adult. Genetically-mediated adaptations, such as variations in the pigmentation of our skin, have ensured our evolutionary fitness across all latitudes.
Will the human species continue to adjust and adapt to new environmental challenges in the future? If past performance is any measure of future expectations, then the human story will continue as long as we do not alter our environment to the point that the plasticity of our behavior, physiological, and morphological boundaries is exceeded.
Misconceptions About Human Evolution
- There is no separation of culture from science, or facts from values, in human evolution.
As we have seen, the scientific study of who we are and where we come from is not biology. It is a branch of anthropology that overlaps in crucial ways with biology, and yet it also traffics in the world of politics, cultures, moral codes, and histories. This is not to say that other sciences can necessarily be free of culture but simply that it is easier to be objective about boron than about your ancestors. Narratives about ancestors are invariably sacred stories, and biological anthropologists incur an unusual responsibility in being the scientific custodians of our ancestors’ stories-writing and validating their stories, shepherding them through history.
- Equality is not identity.
The great geneticist Theodosius Dobzhansky emphasized the distinction between equality (a political state) and identity (a biological state). Sameness/difference is unrelated to equal/unequal, under our system of government. No matter what kind of person you are, you are entitled to equality. Consequently all discussions of race or sex are irrelevant to questions of rights: All citizens are entitled to equal rights. The difficulty is how to guarantee that all receive them, which is a political issue, because obviously there is a great deal of inequality in America. Patterns of social inequality are not grounded in human biological variation. It has become a moral challenge for the nation and for science to better understand this fact, particularly as critiques of equality are too often accompanied by pseudo-biological arguments.
- All humans are equally close to apes, despite the attempt of some people to question the essential humanity of certain populations by suggesting that some people are more apelike than others.
The suggestion that some groups of humans are more naturally apelike than others is a recurrent slander of the modern age. Apelike is obviously a synonym for subhuman; and the symbolic association of apes with African peoples is actually a pre-Darwinian slur, from centuries before evolutionary theory was developed. All humans are equally distantly related from the chimpanzee, but some humans, especially people of color, have been symbolically dehumanized throughout modern history by associating them with apes. Consequently, such a comparison is no longer considered funny.
Terms You Should Know
Inheritance of Acquired Characteristics
Study Questions
- Detail at least two examples of how natural selection has influenced human variation. Specifically, what was the selective pressure that may have led to a preference for a specific trait and how is that trait related to an increased level of fitness?
- What was gained by reducing organisms to genotypes and species to gene pools? What is gained by reintroducing bodies and species into evolutionary studies?
- How are you reducible to your ancestry? If you could meet your ancestors from the year 1700 (and you would have well over a thousand of them!), would their lives be meaningfully similar to yours? Would you even be able to communicate with them?
- Detail at least two examples of how natural selection has influenced human variation. Specifically, what was the selective pressure that may have led to a preference for a specific trait and how is that trait related to an increased level of fitness?
References
Al-Jahiz. 776–868 CE. Kitab al-Hayawan (Book of Animals).
Bigham, Abigail W. 2016. Genetics of Human Origin and Evolution: High-Altitude Adaptations. Current Opinion in Genetics & Development 41: 8–13.
Cyrkalff, Marek, Cecilia P. Sanchez, Nicole Kilian, Curille Bisseye, Jacques Simpore, Friedrich Frischknecht, and Michael Lanzer. 2011. Hemoglobins S and C Interfere with Actin Remodeling in Plasmodium falciparum-Infected Erythrocytes. Science 334 (6060): 1283–1286.
Darwin, Charles. 1859. On the origin of species by means of natural selection, or, the preservation of favoured races in the struggle for life. London: J. Murray.
Dzialanski, Zbigniew, Michael Barany, Peter Engfeldt, Anders Magnuson, Lovisa A. Olsson, and Torbjӧrn K. Nilsson. 2016. Lactase Persistence versus Lactose Intolerance: Is There an Intermediate Phenotype? Clinical Biochemistry, 49(2016): 248–252.
Fernando, Sumadya D., Chaturaka Rodrigo, and Senaka Rajapakse. 2010. The ‘Hidden’ Burden of Malaria: Cognitive Impairment Following Infection. Malaria Journal, 9(366): 1–11.
Haeckel, Ernst. 1868. Natürliche Schöpfungsgeschichte. Berlin: Reimer.
Hartman, T. K., S. J. Rogerson, and P. R. Fischer. 2013. The Impact of Maternal Malaria on Newborns. Annals of Tropical Paediatrics, 30(4): 271–282.
Linnaeus, Carl. 1736. Systema Naturae. First Edition. Stockholm: Laurentius Salvius.
Longo, Dan L., Frédéric B. Piel, Martin H. Steinberg, and David C. Rees. 2017. Sickle Cell Disease. The New England Journal of Medicine 376(16): 1561–1573.
Meyer, M. C., M. S. Alexander, Z. Wang, D. L. Hoffmann, J. A. Dahl, D. Degering, W. R. Haas, and F. Schlütz. 2017. “Permanent Human Occupation of the Central Tibetan Plateau in the Early Holocene.” Science 355 (6320): 64–67.
World Health Organization. 2023. Malaria.
A Derivative Work From
Cochrane, Logan. 2020. Seeing Like an Anthropologist: Anthropology in Practice. In Brown, Nina et al. (eds.), Perspectives, an Open Introduction to Cultural Anthropology, 2nd Edition. American Anthropological Association
Shook, Beth; Nelson, Katie; Aguilera, Kelsie; and Braff, Lara. 2023. Explorations: An Open Invitation to Biological Anthropology, Second Edition. American Anthropological Association
How groups of species are organized and classified based on how they look and how they are related to each other.
A system of grouping organisms according to a hierarchy, or levels.
The study of physical correspondences among diverse kinds of creatures; necessary for creating taxonomies
A fit between the organism and environment.
The idea that all people share a common single origin.
The idea that different peoples are fundamentally different, with different origins, and have always been so.
Darwin’s term for what we now call “evolution,” in which animals and plants derive from but look different from their ancestors.
A consistent bias in survival and fertility, leading to the over-representation of certain features in future generations and an improved fit between an average member of the population and the environment.
The idea that you pass on the features that developed during your lifetime, not just your genes; also known as Lamarckian inheritance.
A genetic variant.
A stretch of DNA with an identifiable function (sometimes broadened to include any DNA with recognizable structural features as well).
The genetic constitution of an individual organism
The hypothetical summation of the entire genetic composition of a population or species.
Explains the evolution of life in terms of genetic changes occurring in the population that leads to the formation of new species.
The observable manifestation of a genetic constitution, expressed in a particular set of circumstances; an organism's physical appearance.
Random, short-term perturbations to the gene pool, with nonadaptive effects.
The geographical movement of genes, due to the contact of populations.
An alteration to the base sequence of DNA.
Chains of amino acids that fold into a three-dimensional structure that allow a cell to function in a variety of ways.
Fatty acid molecules that serve various purposes in the cell, including energy storage, cell signaling, and structure.
Molecules composed of carbon and hydrogen atoms that can be broken down to supply energy.
Complex structures (like DNA or RNA) that carry genetic information about a living organism.
A molecule that carries the hereditary information passed down from parents to offspring. DNA can be described as a “double helix”’ shape. It includes two chains of nucleotides held together by hydrogen bonds with a sugar phosphate backbone.
The order of nucleotide bases. A DNA sequence can be short, long, or representative of entire chromosomes or organismal genomes.
Single-stranded nucleic acid molecule. There are different RNAs found within cells and they perform a variety of functions, such as cell signaling and involvement in protein synthesis.
Chemical bonding between nucleotides. In DNA, adenine (A) pairs with thymine (T) and cytosine (C) pairs with guanine (G); in RNA, adenine (A) always pairs with uracil (U).
A DNA molecule that is wrapped around protein complexes, including histones.
Cellular process in which DNA is copied and doubled.
A cycle the cell undergoes with checkpoints between phases to ensure that DNA replication and cell division occur properly.
Proteins responsible for catalyzing (accelerating) various biochemical reactions in cells.
A protein that breaks the hydrogen bonds that hold double-stranded DNA together.
Enzymes that add nucleotides to existing nucleic acid strands during DNA replication. These enzymes can be distinguished by their processivity (e.g., DNA replication).
Organic molecules that are the building blocks of protein. Each of the 20 different amino acids have their own unique chemical property. Amino acids are chained together to form proteins.
A multi-step process by which amino acids are strung together by RNA machinery read from a DNA template.
RNA molecule that is transcribed from DNA. Its tri-nucleotide codons are “read” by a ribosome to build a protein.
The process by which mature mRNAs are produced during protein synthesis. Introns are removed (spliced) and exons are joined together.
An organelle in the cell found in the cytoplasm or endoplasmic reticulum. It is responsible for reading mRNA and protein assemblage.
Genetically mediated, non-environmentally influenced base melanin level.
Increase in melanin production in response to ultraviolet radiation (UV) exposure.
A pattern of gradual and continuous variation in a physical trait across a geographic area
Form of B complex vitamin necessary for proper fetal development.
For mammals of the same species, those with more darkly pigmented forms tend to be found closer to the equator and those with lighter forms are found in regions further from the equator.
For a broadly distributed monophyletic group, species and populations of smaller size tend to be found in environments with warmer climates and those of larger size tend to be found in ones that are colder.
Due to thermal adaptation, homeothermic animals have body volume-to-surface ratios that vary inversely with the average temperature of their environment. In cold climates, the anticipated ratio is high; in warm climates, it is low.
A type of infectious disease naturally transmitted between animals and humans.
A group of genetically inherited blood disorders characterized by an abnormality in the shape of the hemoglobin within erythrocytes (red blood cells).
Genetic mutation permitting the continued production of lactase-phlorizin hydrolase enzyme in the small intestine past the weaning period.
Evolutionary process whereby organisms that are not closely related independently evolve similar traits as a product of adaptation to similar evolutionary parameters.