26
Learning Goals
By the end of this reading you should be able to:
- Identify the adaptations to life on land that appear in seedless vascular plants
- Describe the classes of seedless tracheophytes
- Compare the lifecycle of seedless nonvascular and seedless vascular plants
- Explain the role of seedless plants in the ecosystem
Introduction
The vascular plants (also called tracheophytes) are the dominant and most conspicuous group of land plants. The 260,000+ species of tracheophytes compose greater than 90 percent of Earth’s vegetation. Several evolutionary innovations explain their success and their ability to spread to all habitats.
Bryophytes may have been successful at the transition from an aquatic habitat to land, but they are still dependent on water for reproduction and absorb moisture and nutrients through the gametophyte surface. The lack of roots for absorbing water and minerals from the soil, as well as a lack of reinforced conducting cells, limits bryophytes to small sizes. Although they may survive in reasonably dry conditions, they cannot reproduce and expand their habitat range in the absence of water. Vascular plants, on the other hand, can achieve enormous heights, thus competing successfully for light. In this group, photosynthetic organs evolved to become leaves, and pipe-like cells evolved into vascular tissues that transport water, minerals, and fixed carbon throughout the organism.
One group of tracheophytes is the seedless vascular plants, in which the diploid sporophyte is the dominant phase of the lifecycle. The gametophyte in this group is an inconspicuous, but still independent, organism. Throughout plant evolution, there is a shift of roles in the dominant phase of the lifecycle; gametophytes in the non-vascular plants to sporophytes in the vascular plants. Like nonvascular plants, the seedless vascular plants still depend on water during fertilization, as the sperm must swim on a layer of moisture to reach the egg. This characteristic limits both of the groups to mainly moist environments.
Adaptations for Life on Land
Xylem
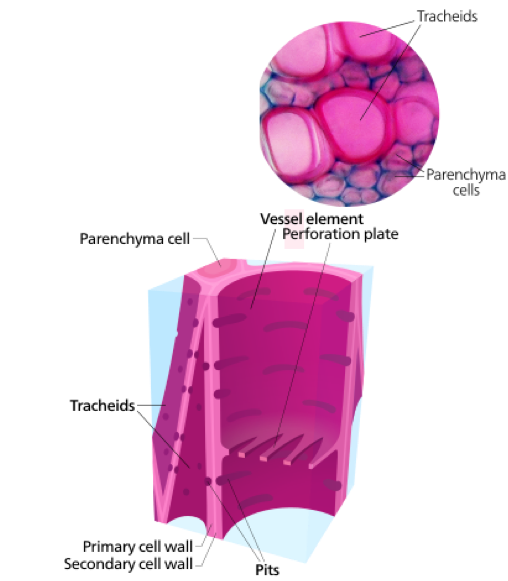
Xylem (Fig. 1) is the tissue responsible for the storage and long-distance transport of water and nutrients, as well as the transfer of water-soluble growth factors from the organs of synthesis to the target organs. The tissue consists of conducting cells, known as tracheids, and supportive filler tissue called parenchyma. When xylem cells are mature they are dead, meaning that the internal membrane and structures are lost, leaving just an empty tube. Xylem conductive cells incorporate the compound lignin into their walls and are thus described as lignified. Lignin is a complex polymer that is impermeable to water which “seals’ the dead xylem cells and confers mechanical strength to vascular tissue. With their rigid cell walls, xylem cells provide support to the plant and allow the plant to grow upwards against the pull of gravity. Taller plants often have a selective advantage by being able to reach unfiltered sunlight and disperse their spores or seeds further away, thus expanding their range. By growing higher than other plants, tall er plants cast their shadow on shorter plants and limit competition for light, water, and precious nutrients in the soil.
Phloem
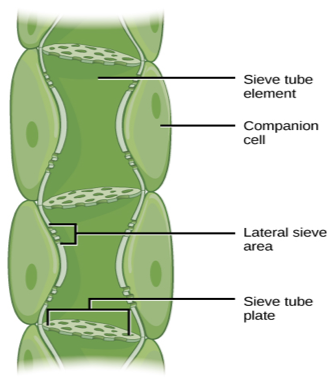
Phloem (Fig. 2) is the second type of vascular tissue; it transports sugars, proteins, and other solutes throughout the plant. Phloem cells are divided into sieve elements (conducting cells) and cells that support the sieve elements (companion cells). Unlike xylem, phloem consists of living cells that are connected by highly developed pores. In order to aid in the transport of materials, mature conducting cells often lack a nucleus and other internal organelles and must rely on nearby cells for needed proteins. Without the internal organelles, the flow of materials is unimpeded and therefore more efficient.
Roots
Roots (Fig. 3) are not well preserved in the fossil record. Nevertheless, it seems that roots appeared later in evolution than vascular tissue. The development of an extensive network of roots represented a significant new feature of vascular plants. Thin rhizoids attached mosses (nonvascular plants) to the substrate, but these rather flimsy filaments did not provide a strong anchor for the plant; neither did they absorb substantial amounts of water and nutrients.
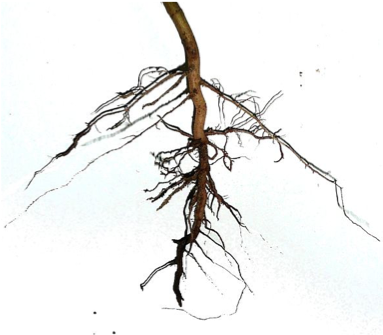
In contrast, roots, with their prominent vascular tissue system, transfer water and minerals from the soil to the rest of the plant. The extensive network of roots that penetrates deep into the soil to reach sources of water also stabilizes taller plants by acting as a ballast or anchor.
The majority of roots establish a symbiotic relationship with fungi, forming mycorrhizae, which benefit the plant by greatly increasing the surface area for absorption of water and soil minerals and nutrients.
Leaves, Sporophylls, and Strobili
A third innovation marks the seedless vascular plants. Accompanying the prominence of the sporophyte and the development of vascular tissue, the appearance of true leaves improved their photosynthetic efficiency. Leaves capture more sunlight with their increased surface area by employing more chloroplasts to trap light energy and convert it to chemical energy, which is then used to fix atmospheric carbon dioxide into carbohydrates. The carbohydrates are exported to the rest of the plant by the conductive cells of phloem tissue.
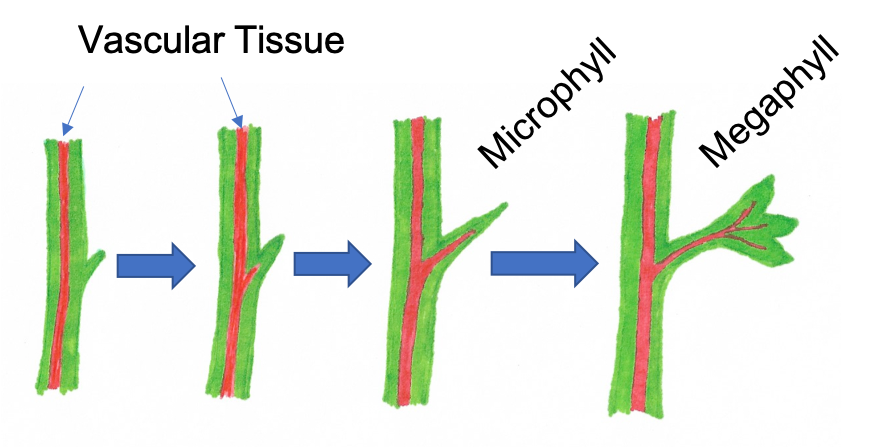
The existence of two types of morphology suggests that leaves evolved independently in several groups of plants. The first type of leaf is the microphyll, or “little leaf,” which can be dated to 350 million years ago. A microphyll is small and has a simple vascular system. A single unbranched vein—a bundle of vascular tissue made of xylem and phloem—runs through the center of the leaf. Microphylls may have originated from the flattening of lateral branches, or from sporangia that lost their reproductive capabilities (Fig. 4). Microphylls are present in the club mosses and probably preceded the development of megaphylls, or “big leaves”, which are larger leaves with a pattern of branching veins. Megaphylls most likely appeared independently several times during the course of evolution. Their complex networks of veins suggest that several branches may have combined into a flattened organ, with the gaps between the branches being filled with photosynthetic tissue.
In addition to photosynthesis, leaves play another role in the life of plants. Pine cones, mature fronds of ferns, and flowers are all sporophylls—leaves that were modified structurally to bear sporangia. Strobili are cone-like structures that contain sporangia. They are prominent in conifers and are commonly known as pine cones.
Review Question:
By the late Devonian period, plants had evolved vascular tissue, well-defined leaves, and root systems. With these advantages, plants increased in height and size. During the Carboniferous period, swamp forests of club mosses and horsetails—some specimens reaching heights of more than 30 m (100 ft)—covered most of the land. These forests gave rise to the extensive coal deposits that gave the Carboniferous its name. In seedless vascular plants, the sporophyte is the dominant phase of the lifecycle. Water is still required for the fertilization of seedless vascular plants, and most favor a moist environment. Modern-day seedless tracheophytes include club mosses, horsetails, ferns, and whisk ferns.
Phylum Lycopodiophyta: Club Mosses, quillworts and spike mosses
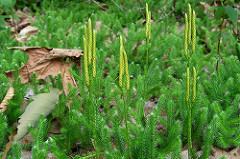
The Lycophytes, which are not true mosses, are the earliest group of seedless vascular plants. They dominated the landscape of the Carboniferous, growing as tall as trees and forming large swamp forests. Today’s club mosses. however, are small, evergreen plants consisting of a stem (which may be branched) and microphylls. The phylum Lycopodiophyta consists of close to 1,200 species, including the quillworts (Isoetales), the club mosses (Lycopodiales) (Fig. 5), and spike mosses (Selaginellales) (Fig. 6).
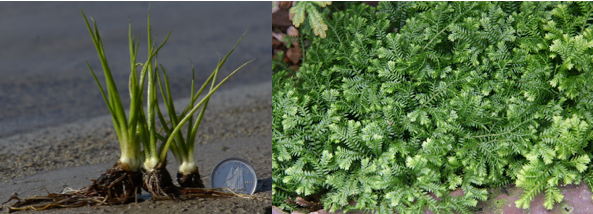
Lycophytes display an alternation of generations in which the sporophyte is the major stage of the lifecycle. In addition, the gametophytes grow separately and thus do not depend on the sporophyte for nutrients. There are some species of Lycophyte in which the gametophytes develop underground and form mycorrhizal associations with fungi. Lycophyte species can be homosporous, producing only one type of spore or heterosporous, producing two different types of spores. In club mosses, the sporophyte gives rise to sporophylls arranged in strobili, cone-like structures that give the class its name.
Phylum Monilophyta: Class Equistopsida (Horsetails)
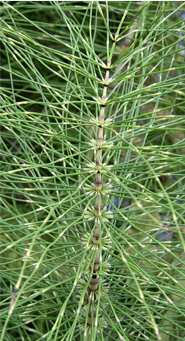
Horsetails (Fig. 7), whisk ferns and ferns belong to the phylum Monilophyta, with horsetails placed in the Class Equisetopsida. There is only a single genus Equisetum that is the survivor of a large group of plants, known as Arthrophyta. During the Carboniferous period this group, like the Lycophytes, produced large plants and entire swamp forests. Today’s Equisetum(Horsetail) plants are usually found in damp environments and marshes and have a unique morphology. The stem of a horsetail is characterized by the presence of joints or nodes, hence the name Arthrophyta (arthro- = “joint”; -phyta = “plant”). Modified leaves and branches come out as whorls from the evenly spaced joints. The needle-shaped leaves do not contribute greatly to photosynthesis, the majority of which takes place in the green stem. Another unique feature is that silica collects in the epidermal cells of these plants, contributing to the stiffness of horsetail plants. Underground stems are known as rhizomes anchor the plants to the ground. Modern-day horsetails are homosporous, producing bisexual gametophytes.
Phylum Monilophyta: Class Psilotopsida (Whisk Ferns)
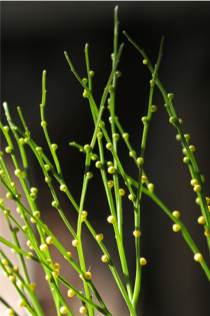
While most ferns form large leaves and branching roots, the whisk ferns (Fig. 8), Class Psilotopsida, lack both roots and leaves. Photosynthesis takes place in their green stems, while water and mineral absorption occur in the horizontal underground rootlike stems (rhizomes)that have mycorrhizal associations with fungi. Small yellow knobs form at the tip of the branch stem and contain the sporangia. There are two phases in the life cycle of a whisk fern. The large asexual plants (sporophytes) produce spores that develop into very small colorless gametophytes, which are similar to rhizomes in overall appearance. Eggs and sperm are produced in special structures on the gametophyte. The union of these gametes initiates the sporophyte phase. Whisk ferns were considered an early pterophyte. However, recent comparative DNA analysis suggests that this group may have lost both leaves and roots through evolution, and are most closely related to ferns.
Phylum Monilophyta: Class Psilotopsida (Ferns)
With their large fronds, ferns (Fig. 9) are the most readily recognizable seedless vascular plants. They are considered the most complex seedless vascular plants and display characteristics commonly observed in seed plants. 20,000+ species of ferns live in environments ranging from tropics to temperate forests. Although some species survive in dry environments, most ferns are restricted to moist, shaded places. Ferns made their appearance in the fossil record during the Devonian period and expanded during the Carboniferous.
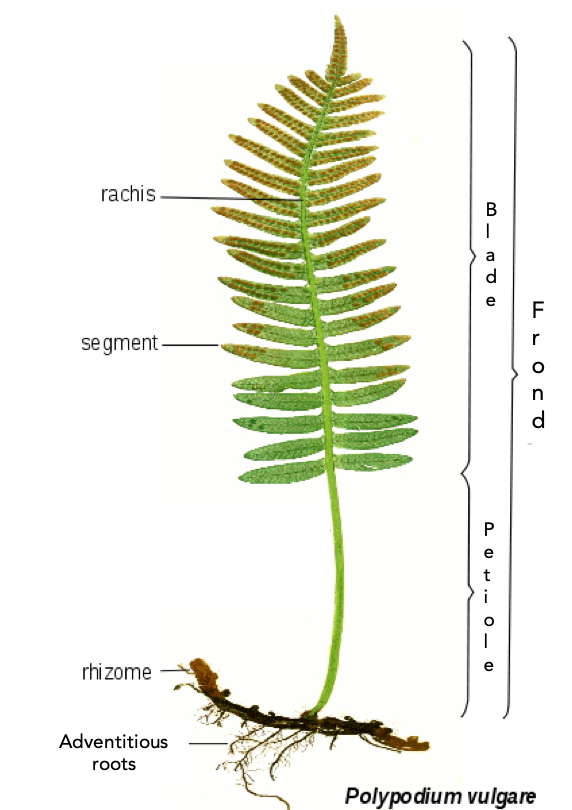
The dominant stage of the lifecycle of a fern is the sporophyte, which consists of large compound leaves called fronds. Fronds fulfill a double role; they are photosynthetic organs that also carry reproductive organs. The stem may be buried underground as a rhizome, from which adventitious roots grow to absorb water and nutrients from the soil; or, they may grow above ground as a trunk in tree ferns. Adventitious organs are those that grow in unusual places, such as roots growing from the side of a stem.
In some fern species, the tip of a developing fern frond is rolled into a crozier or fiddlehead. Fiddleheads unroll as the frond develops. Most ferns produce the same type of spores and are therefore homosporous. The diploid sporophyte is the most conspicuous stage of the lifecycle. On the underside of its mature fronds, sori (singular, sorus) form as small clusters where sporangia develop (Fig. 10). Inside the sori, spores are produced by meiosis and released into the air.
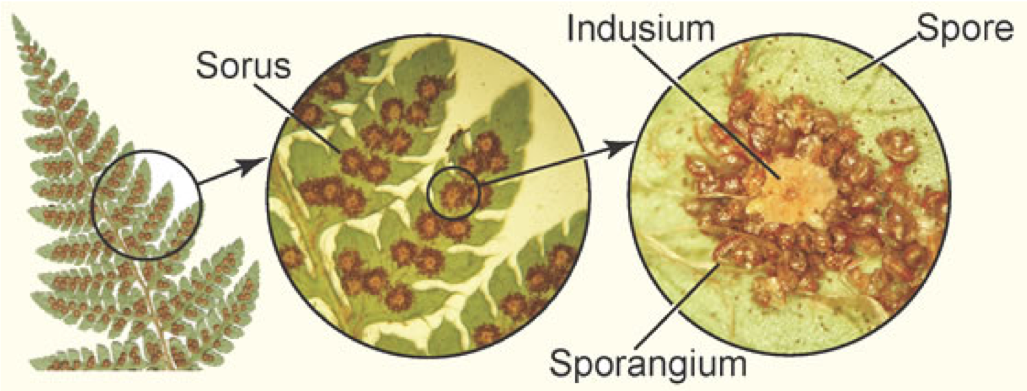
Those that land on a suitable substrate germinate and form a heart-shaped gametophyte, which is attached to the ground by thin filamentous rhizoids. The inconspicuous gametophyte harbors both sex gametangia. Flagellated sperm released from the antheridium swim on a wet surface to the archegonium, where the egg is fertilized. The newly formed zygote grows into a sporophyte that emerges from the gametophyte and grows by mitosis into the next generation sporophyte. Each gametophyte only produces one sporophyte but each sporophyte produces millions of spores.
Summary
Vascular systems consist of xylem tissue, which transports water and minerals, and phloem tissue, which transports sugars and proteins. With the development of the vascular system, there appeared leaves to act as large photosynthetic organs, and roots to access water from the ground. Small uncomplicated leaves are microphylls. Large leaves with vein patterns are megaphylls. Modified leaves that bear sporangia are sporophylls. Some sporophylls are arranged in cone structures called strobili.
The seedless vascular plants include club mosses, which are the most primitive; whisk ferns, which lost leaves and roots by reductive evolution; and horsetails and ferns. Ferns are the most advanced group of seedless vascular plants. They are distinguished by large leaves called fronds and small sporangia-containing structures called sori, which are found on the underside of the fronds.
The seedless plants (nonvascular and vascular) play an essential role in the balance of the ecosystems; they are pioneering species that colonize bare or devastated environments and make it possible for succession to occur. They contribute to the enrichment of the soil and provide shelter and nutrients for animals in hostile environments.
End of Section Review Questions:
Image Attribution
Figure 1. Image courtesy of Kelvinsong [CC BY-SA 3.0 (https://creativecommons.org/licenses/by-sa/3.0)]
Figure 2. Image courtesy of CNX OpenStax [CC BY 4.0 (https://creativecommons.org/licenses/by/4.0)]
Figure 3. Image courtesy of RoRo [CC0]
Figure 4. Image created and provided by D. Jennings
Figure 5. Image courtesy of Jason Hollinger on flickr [CC BY-SA 2.0 (https://creativecommons.org/licenses/by/2.0/)]
Figure 7. Image courtesy of Tony Hisgett from Birmingham, UK [CC BY 2.0 (https://creativecommons.org/licenses/by/2.0)]
Figure 8. Image courtesy of Mary Keim on Flickr[CC BY 2.0 (https://creativecommons.org/licenses/by/2.0)]
Figure 9. Image courtesy of NuriaWrite [CC BY-SA 3.0 (https://creativecommons.org/licenses/by-sa/3.0)]
Figure 10. Image courtesy of Abalg [CC BY-SA 3.0 (https://creativecommons.org/licenses/by-sa/3.0)] Modified by D. Jennings