3
Learning Goals
-
Describe the different types of point mutations
-
Differentiate between different chromosomal mutations
-
Explain how mutations play a role in evolutionary processes
-
Discuss factors that can influence the rate of mutations
Introduction
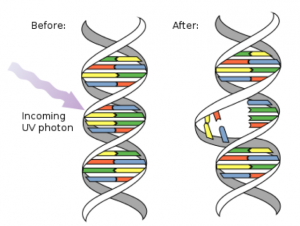
A cell’s DNA is the repository of all the information needed to not only make the cell but to enable it to function. In addition, the chromosome(s) also contain the information to make any cell in the organism. Mutations are variations that occur in the DNA sequence which alter the genetic code and can be passed on through cell division (Fig. 1). It is the genetic variation within cells and within individuals that account in part for the physical differences that we see between individuals and between groups of organisms. Genetic variation is responsible for the diversity of organisms that are on this planet, from single-celled bacteria to the largest animal, the blue whale. This variation is a key factor in the ability of populations to evolve and ultimately adapt to their environments.
Mutations are the ultimate source of genetic variation
Small Scale Mutations
Mutations can also be the result of the addition of a base, known as an insertion, or the removal of a base, also known as deletion. These types of mutations can alter the reading frame (3 nucleotides code for 1 amino acid) of the amino acids, and thus the sequence of amino acids that are incorporated into the protein. The number of nucleotides that are inserted or deleted will determine how much of a change, and thereby how damaging the mutation is. If fewer than 3 insertions or deletions occur in a row, then a frameshift mutation can occur. This alters the reading of the triplet codons during translation of mRNA to proteins and most often results in a non-functional protein. Finally, sometimes a piece of DNA from one chromosome may get translocated to another chromosome or to another region of the same chromosome; this is also known as translocation.
Review Question:
Chromosomal Mutations
Smaller-scale duplications that include only one or a few genes can be maintained over many generations depending on the genes that are involved. Deletions can occur as a result of an error in replication or from the joining of breaks in a chromosome that eliminates a section. In some cases, deletions can persist in a population because chromosomes occur in homologous pairs in diploid organisms. This means that a copy of the deleted region is still present in one of the homologs and one copy may be enough for survival and reproduction. Some deletions, on the other hand, decrease the chance of survival and/or reproduction even when the homologous chromosome is normal.
Chromosomal Inversions and Translocations
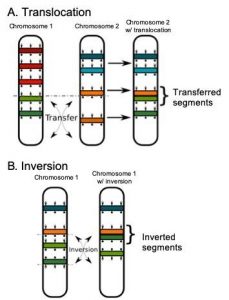
In addition to duplications and deletions, the order of genes within a chromosome can also be altered through inversions and reciprocal translocations. An inversion occurs when a block of genes within a chromosome is reversed, typically when a segment between two breaks and the segment is flipped in orientation. In larger genomes, these breaks are most likely to occur in noncoding regions rather than within a gene. Small inversions are common in many populations and because they do not delete or duplicate genes and so are less likely to cause problems. The accumulation of inversions over time long periods of time can explain, at least in part, why the order of genes can differ among closely related organisms.
Reciprocal translocations involve the exchange of segments of genes between two nonhomologous chromosomes. This occurs when there are breaks in both chromosomes and the terminal segments are exchanged before the breaks are repaired. As with inversions, reciprocal translocations change only the arrangement of genes and not their number. Thus, most of these types of mutations do not impact the survival of the organism. However, problems can arise during meiosis as the two chromosomes involved may not pair correctly with their homologs and thus not move properly into the daughter cells. This can result in inequality in gene dosages in the resulting cells, with some cells have duplicates and other missing genes.
Review Question:
Mutation Rates
The most common type of mutation is the substitution of one nucleotide for another but even these types of mutations are relatively rare. The rate of mutations varies among different organisms as well as among different individuals within a population. Multiple factors can influence the rate of mutation and thus mutations rates can also vary over a large range. For example, RNA viruses and retroviruses store their genetic information in RNA rather than DNA. RNA is a less stable molecule than DNA and thus more susceptible to mutations. This means that mutation rates in these viruses are often higher than in viruses that utilize DNA as their genetic material.
Another factor that influences mutations is the proofreading mechanisms found within different groups of organisms. The efficiency with which errors are detected as well as the types of DNA repair mechanisms impact the rate of heritable mutations, mutations that are not corrected, and pass on to subsequent cells/offspring. Thus organisms that have highly efficient and complex detection and repair mechanisms tend to demonstrate lower rates of mutational changes in their genomes.
Since errors do occur, even if infrequently, during the replication of DNA, the mutation rate can vary between individuals within a population. For example, in male mammals, germ line cells undergo meiosis at a much higher rate than in females. This means that the DNA is replicated during each meiotic division and thus since replication occurs more often, the possibility of mutations also increases. The same holds true for different bacteria. Those that are in favorable environments will divide more often than those in less favorable conditions, meaning that their DNA will be replicated more often and thus there is a higher potential for mutations to occur.
Summary
End of Section Review Questions:
1) silent | A) change in one amino acid |
2) missense | B) an insertion/deletion alters the sequence |
3) nonsense | C) a stop code is created |
4) frameshift | D) no change in the resulting protein |
Mutation Characteristics
2) Most mutations are? (Multiple Answers)
Mutation Rates
3) Which of the following has an impact on the rate at which mutations occur in cells? (Multiple Answers)
A) the type of environmental change
B) the number of times DNA is replicated
Review: Think about this
4) Why would a mutation that involves a centromere have a greater impact than a mutation that occurs elsewhere on a chromosome?
References
Adapted from Morris et al. How Life Works 2nd edition (2017) Chapter 14 Mutation and DNA Repair.
Image AttributionFigure 1. Image (UV mutation of DNA) courtesy of derivative work: Mouagip (talk) DNA_UV_mutation.gif: NASA/David Herring. This W3C-unspecified vector image was created with Adobe Illustrator [Public domain]
Figure 2. Image (Point mutations) courtesy of CNX OpenStax[CC BY 4.0]
Figure 3. Left image courtesy of National Human Genome Research Institute [Public domain], via Wikimedia Commons. Right image (chromosomal deletion) was originally uploaded by Mirmillon at French Wikipedia. [Public domain], via Wikimedia Commons
Figure 4. Image courtesy of Guy Leonard / CC BY-SA 3.0 modified by D. Jennings 8/2020
Learning Goals
By the end of this reading you should be able to:
- Classify fungi into the five major phyla
- Describe each phylum in terms of major representative species and patterns of reproduction
Introduction
The kingdom Fungi contains five major phyla that were established according to their mode of sexual reproduction or using molecular data. Polyphyletic, unrelated fungi that reproduce without a sexual cycle, are placed for convenience in a sixth group called a “form phylum”. Not all mycologists agree with this scheme. Rapid advances in molecular biology and the sequencing of 18S rRNA (a part of RNA) continue to show new and different relationships between the various categories of fungi.
The five true phyla of fungi are the Chytridiomycota (Chytrids), the Zygomycota (conjugated fungi), the Ascomycota (sac fungi), the Basidiomycota (club fungi), and the recently described Phylum Glomeromycota. An older classification scheme grouped fungi that strictly use asexual reproduction into Deuteromycota, a group that is no longer in use.
Note: “-mycota” is used to designate a phylum while “-mycetes” formally denotes a class or is used informally to refer to all members of the phylum.
Chytridiomycota: The Chytrids
The only class in the Phylum Chytridiomycota is the Chytridiomycetes. The chytrids are the simplest and most primitive Eumycota, or true fungi (Fig 1). The evolutionary record shows that the first recognizable chytrids appeared more than 500 million years ago. Like all fungi, chytrids have chitin in their cell walls, and one group of chytrids has both cellulose and chitin in their cell wall. Most chytrids are unicellular, though a few form multicellular organisms. The hyphae of these multicellular chytrids have no septa between cells (they are coenocytic). Chytrids produce gametes and diploid zoospores that swim with the help of a single flagellum.
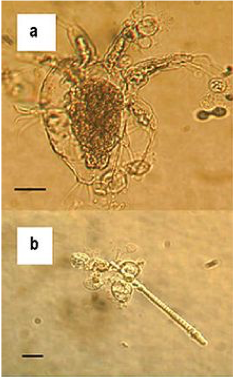
The ecological habitat and cell structure of chytrids have much in common with protists (single-celled eukaryotes that are not considered plants, animals or fungi). Chytrids usually live in aquatic environments, although some species live on land. Some species thrive as parasites on plants, insects, or amphibians, while others are saprobes. The chytrid species Allomyces is well characterized as an experimental organism. Its reproductive cycle includes both asexual and sexual phases. Allomyces produces diploid or haploid flagellated zoospores in a sporangium.
Zygomycota: The Conjugated Fungi
The zygomycetes are a relatively small group of fungi belonging to the Phylum Zygomycota. They include the familiar bread mold, Rhizopus stolonifer, which rapidly propagates on the surfaces of bread, fruits, and vegetables. Most species are saprobes, living off decaying organic material; a few are parasites, particularly of insects. Zygomycetes play a considerable commercial role. The metabolic products of other species of Rhizopus are intermediates in the synthesis of semi-synthetic steroid hormones.
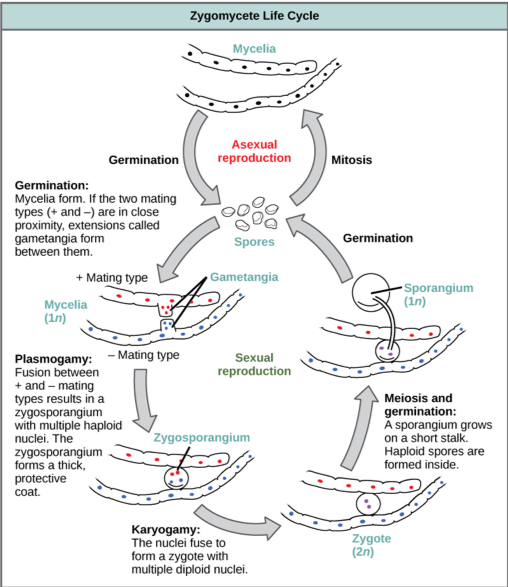
Zygomycetes have a thallus of coenocytic hyphae in which the nuclei are haploid when the organism is in the vegetative stage. The fungi usually reproduce asexually by producing sporangiospores (Fig. 2). The black tips of bread mold are the swollen sporangia packed with black spores (Fig. 3). When spores land on a suitable substrate, they germinate and produce a new mycelium. Sexual reproduction starts when conditions become unfavorable. Two opposing mating strains (type + and type –) must be in close proximity for gametangia from the hyphae to be produced and fuse, leading to karyogamy. The developing diploid zygospores have thick coats that protect them from desiccation and other hazards. They may remain dormant until environmental conditions are favorable. When the zygospore germinates, it undergoes meiosis and produces haploid spores, which will, in turn, grow into a new organism. This form of sexual reproduction in fungi is called conjugation (although it differs markedly from conjugation in bacteria and protists), giving rise to the name “conjugated fungi”.

Ascomycota: The Sac Fungi
The majority of known fungi belong to the Phylum Ascomycota, which is characterized by the formation of an ascus (plural, asci), a sac-like structure that contains haploid ascospores. Many ascomycetes are of commercial importance. Some play a beneficial role, such as the yeasts used in baking, brewing, and wine fermentation, plus truffles and morels, which are held as gourmet delicacies. Aspergillus oryzae is used in the fermentation of rice to produce sake. Other ascomycetes parasitize plants and animals (Fig. 4), including humans. For example, fungal pneumonia poses a significant threat to AIDS patients who have a compromised immune system. Ascomycetes not only infest and destroy crops directly but also produce poisonous secondary metabolites that make crops unfit for consumption. Filamentous ascomycetes produce hyphae divided by perforated septa, allowing the streaming of cytoplasm from one cell to the other. Conidia and asci, which are used respectively for asexual and sexual reproductions, are usually separated from the vegetative hyphae by blocked (non-perforated) septa.
Asexual reproduction is frequent and involves the production of conidiophores that release haploid conidiospores (Fig. 5). Sexual reproduction starts with the development of special hyphae from either one of two types of mating strains (Fig. 5). The “male” strain produces an antheridium and the “female” strain develops an ascogonium. At fertilization, the antheridium and the ascogonium combine in plasmogamy without nuclear fusion. Special ascogenous hyphae arise, in which pairs of nuclei migrate: one from the “male” strain and one from the “female” strain. In each ascus, two or more haploid ascospores fuse their nuclei in karyogamy. During sexual reproduction, thousands of asci fill a fruiting body called the ascocarp. The diploid nucleus gives rise to haploid nuclei by meiosis. The ascospores are then released, germinate, and form hyphae that are disseminated in the environment and start new mycelia.

Review Question:
Basidiomycota: The Club Fungi
The fungi in the Phylum Basidiomycota are easily recognizable under a light microscope by their club-shaped fruiting bodies called basidia (singular, basidium), which are the swollen terminal cell of a hypha. The basidia, which are the reproductive organs of these fungi, are often contained within the familiar mushroom, commonly seen in fields after rain, on the supermarket shelves, and growing on your lawn (Fig. 6). These mushroom-producing basidiomycetes are sometimes referred to as “gill fungi” because of the presence of gill-like structures on the underside of the cap. The “gills” are actually compacted hyphae on which the basidia are borne. This group also includes shelf fungus, which clings to the bark of trees like small shelves. In addition, the Basidiomycota includes smuts and rusts, which are important plant pathogens; toadstools, and shelf fungi stacked on tree trunks. Most edible fungi belong to the Phylum Basidiomycota; however, some basidiomycetes produce deadly toxins. For example, Cryptococcus neoformans causes severe respiratory illness.

The lifecycle of basidiomycetes includes alternation of generations (Fig. 7). Spores are generally produced through sexual reproduction, rather than asexual reproduction. The club-shaped basidium carries spores called basidiospores. In the basidium, nuclei of two different mating strains fuse (karyogamy), giving rise to a diploid zygote that then undergoes meiosis. The haploid nuclei migrate into basidiospores, which germinate and generate monokaryotic hyphae. The mycelium that results is called a primary mycelium. Mycelia of different mating strains can combine and produce a secondary mycelium that contains haploid nuclei of two different mating strains. This is the dikaryotic stage of the basidiomycetes life cycle and it is the dominant stage. Eventually, the secondary mycelium generates a basidiocarp, which is a fruiting body that protrudes from the ground—this is what we think of as a mushroom. The basidiocarp bears the developing basidia on the gills under its cap.

Review Question:
Thinking Question:
Asexual Ascomycota and Basidiomycota
Imperfect fungi—those that do not display a sexual phase—use to be classified in the form phylum Deuteromycota, a classification group no longer used in the present, ever-developing classification of organisms. While Deuteromycota used to be a classification group, recent molecular analysis has shown that the members classified in this group belong to the Ascomycota or the Basidiomycota classifications. Since they do not possess the sexual structures that are used to classify other fungi, they are less well described in comparison to other members. Most members live on land, with a few aquatic exceptions. They form visible mycelia with a fuzzy appearance and are commonly known as mold.
The reproduction of the fungi in this group is strictly asexual and occurs mostly by the production of asexual conidiospores (Fig. 8). Some hyphae may recombine and form heterokaryotic hyphae. Genetic recombination is known to take place between the different nuclei.

The fungi in this group have a large impact on everyday human life. The food industry relies on them for ripening some cheeses. The blue veins in Roquefort cheese and the white crust on Camembert are the results of fungal growth. The antibiotic penicillin was originally discovered on an overgrown Petri plate, on which a colony of Penicillium fungi killed the bacterial growth surrounding it. Other fungi in this group cause serious diseases, either directly as parasites (which infect both plants and humans), or as producers of potent toxic compounds, as seen in the aflatoxins released by fungi of the genus Aspergillus.
Glomeromycota
The Glomeromycota is a newly established phylum that comprises about 230 species that all live in close association with the roots of trees. Fossil records indicate that trees and their root symbionts share a long evolutionary history. It appears that all members of this family form arbuscular mycorrhizae: the hyphae interact with the root cells forming a mutually beneficial association where the plants supply the carbon source and energy in the form of carbohydrates to the fungus, and the fungus supplies essential minerals from the soil to the plant.
The glomeromycetes do not reproduce sexually and do not survive without the presence of plant roots. Although they have coenocytic hyphae like the zygomycetes, they do not form zygospores. DNA analysis shows that all glomeromycetes probably descended from a common ancestor, making them a monophyletic lineage.
Summary
Chytridiomycota (chytrids) are considered the most primitive group of fungi. They are mostly aquatic, and their gametes are the only fungal cells known to have flagella. They reproduce both sexually and asexually; the asexual spores are called zoospores. Zygomycota (conjugated fungi) produce non-septated hyphae with many nuclei. Their hyphae fuse during sexual reproduction to produce a zygospore in a zygosporangium. Ascomycota (sac fungi) form spores in sacs called asci during sexual reproduction. Asexual reproduction is their most common form of reproduction. Basidiomycota (club fungi) produce showy fruiting bodies that contain basidia in the form of clubs. Spores are stored in the basidia. Most familiar mushrooms belong to this division. Fungi that have no known sexual cycle were classified in the form phylum Deuteromycota, which the present classification puts in the phyla Ascomycota and Basidiomycota. Glomeromycota forms tight associations (called mycorrhizae) with the roots of plants.
End of Section Review Questions:
Review: Reproductive Structure
Review: No Sex
Review: Symbiosis
Review: Decomposition
Review: Symbiosis
Review: Compare
Learning Goals
By the end of this reading you should be able to:
- Identify the two supergroups of Eukarya that contain plants, animals, and fungi
- Explain why the presence of photosynthesis is not used as a defining characteristic of Eukaryotic supergroups
- Compare the traits shared by green algae and land plants
- Explain the reasons why Charales are considered the closest relative to land plants
Introduction
The oldest fossils appear to all be prokaryotes, while the evidence of eukaryotes is about 2 billion years old (they appear later in the fossil record). Based on this it is probable that today’s eukaryotes are descended from an ancestor that had a prokaryotic organization. The last common ancestor of today’s Eukarya had several characteristics, including cells with nuclei that divided mitotically and contained linear chromosomes where the DNA was associated with histones, a cytoskeleton and endomembrane system, and the ability to make cilia/flagella during at least part of its life cycle. This ancestor was aerobic because it had mitochondria that were the result of endosymbiosis of an aerobic alpha-proteobacterium. Whether this ancestral cell had a nucleus at the time of the initial symbiosis remains unknown. Modern Eukarya are an amazingly diverse group of organisms, from single cells to complex multicellular organisms and with multiple means of acquiring both energy and the carbon need for building blocks.
Supergroups within the Eukarya
The emerging classification scheme groups the entire domain Eukaryota into six “supergroups” that evolved from a common ancestor and contains all of the protists as well as animals, plants, and fungi (Fig. 1).
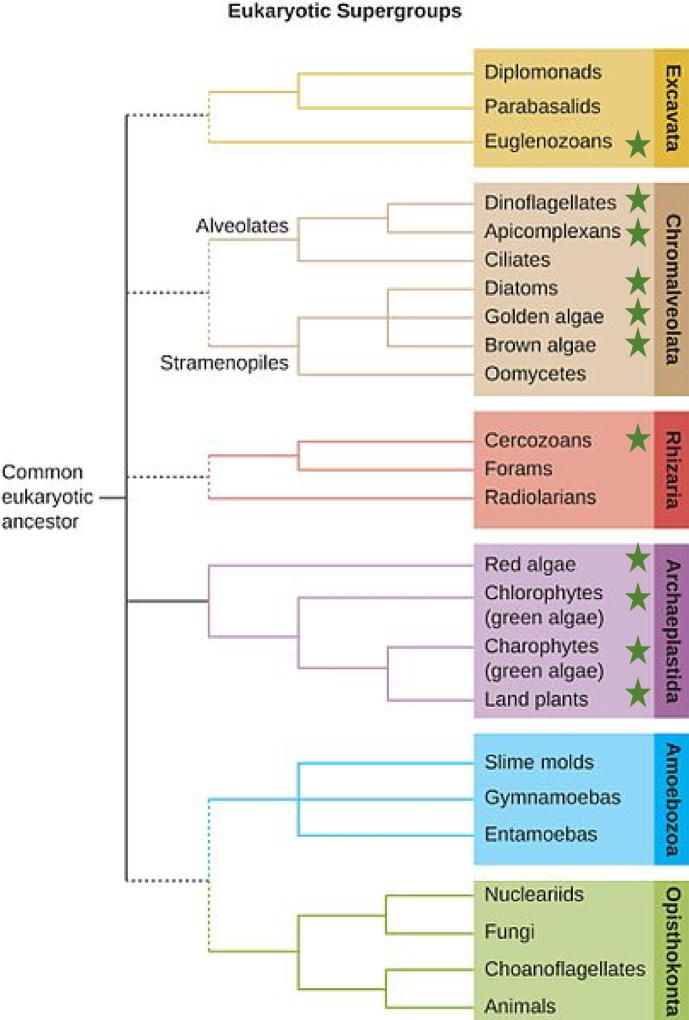
The supergroups are believed to be monophyletic, meaning that all organisms within each supergroup are believed to have evolved from a single common ancestor, and thus all members are most closely related to each other than to organisms outside that group. There is still evidence lacking for the monophyly of some groups.
The classification of eukaryotes is still in flux, and the six supergroups may be modified or replaced by a more appropriate hierarchy as genetic, morphological, and ecological data accumulate. Keep in mind that the classification scheme presented here is just one of several hypotheses, and the true evolutionary relationships are still to be determined.
Archaeplastida
The supergroup Archaeplastida is separated into three phylogenetic groups: the Glaucocytophyes, the Red Algae, and the Viridioplantae. With only a few exceptions all of the organisms within the supergroup are photosynthetic. Photosynthesis, however, is found in other groups that are not within the Archaeplastida, including the brown and golden algae, diatoms, dinoflagellates, some apicomplexans, some excavata (Euglenids), and some Rhizarians (see Fig. 2). Red algae and green algae, however, are included in the supergroup Archaeplastida. Molecular evidence supports that all Archaeplastida are descendants of an endosymbiotic relationship between a heterotrophic protist and a cyanobacterium.
Thinking Question:
What is a possible explanation for the pattern of distribution of photosynthetic eukaryotes (they are widely distributed but discontinuously among the different groups)? Be sure to provide support for your reasoning.
Glaucophytes:
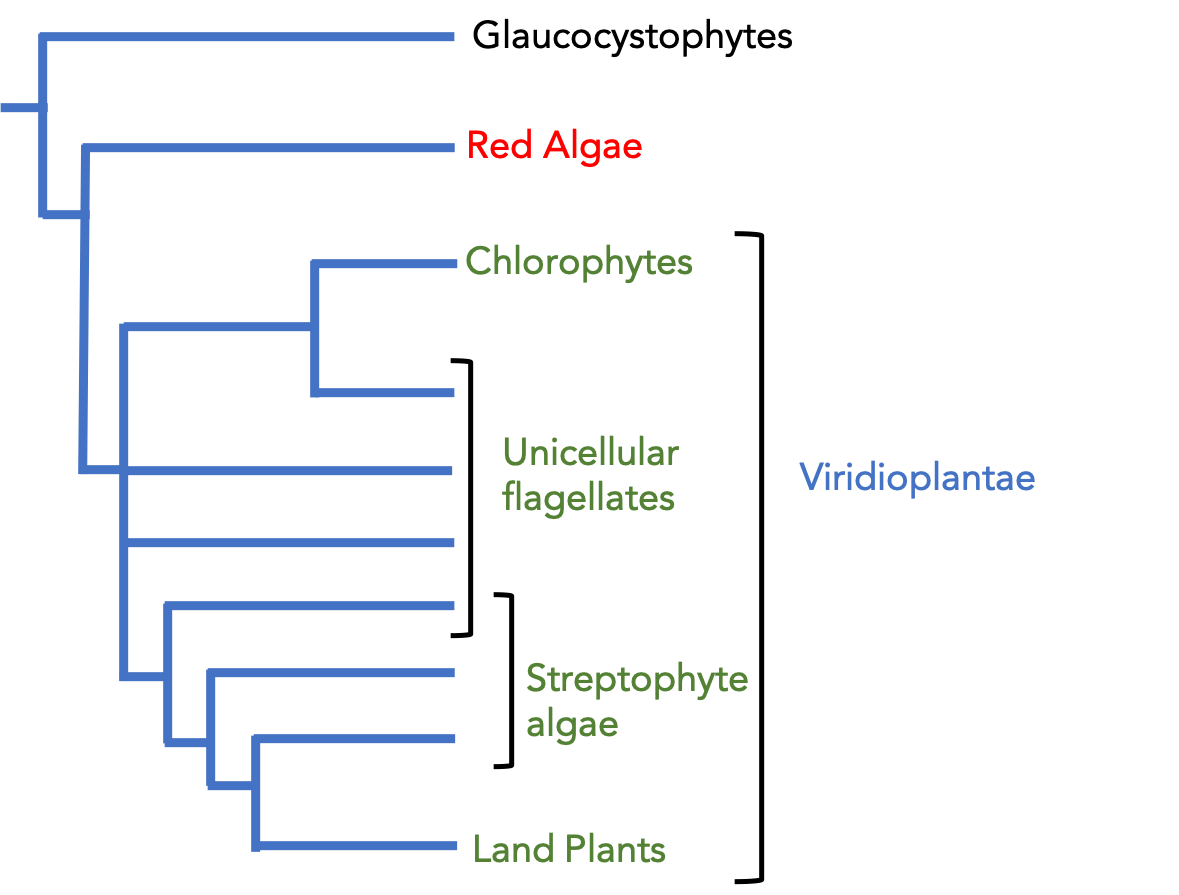
This is a small group of organisms that reside in freshwater ponds and lakes. They are unique in that their chloroplasts have walls of peptidoglycan, a feature retained from the ancestral cyanobacterial endosymbiont. In addition, their photosynthetic pigments include biliprotein which is also found in cyanobacteria.
Red Algae
Red algae, or rhodophytes, are primarily multicellular, most lack flagella, and range in size from microscopic, unicellular protists to large, multicellular forms that have differentiated tissues and in some cases complex morphology. Red algae, like land plants, have cell walls composed of cellulose and display a life cycle with an alternation of generations. There are many species of red algae that are seaweeds that are found in the shallow seafloor, however, and some species have unique pigment photosynthetic accessory pigments. These phycoerythrin pigments are red in color which allows the algae to utilize short (blue) wavelengths of light. These wavelengths are found at greater depths than the red wavelengths commonly used by other photosynthetic organisms and thus allow these algae to live at greater depths and outcompete other algae. Coralline red algae are found at the edges of coral reefs and they have the ability to secrete calcium carbonate (CaCO3) to form an external skeleton-like structure that protects them from the motion of the waves. These tiny red algae are key to the establishment of the coral reefs as they provide a needed buffer for the newly attached corals.
Viridioplantae: Green Algae
Green algae are the most abundant group of algae and exhibit similar features to the land plants. The cells in green algae divide along cell plates called phragmoplasts, and their cell walls are layered in the same manner as the cell walls of land plants. In addition, green algae contain the same carotenoids and chlorophyll a and b as land plants, whereas other algae have different accessory pigments and types of chlorophyll molecules in addition to chlorophyll a. Both green algae and land plants also store carbohydrates like starch. These similarities provide evidence that, even though green algae are classified as protists, they shared a relatively recent common ancestor with land plants.
There is wide diversity within the green algae, and while some groups are unicellular and flagellate, the Chlorophytes and the Charophytes contain members that are complex and multicellular. The Chlorophytes include more than 7000 different species that live in fresh or brackish water, in seawater, or in snow patches. A few even survive on soil, provided it is covered by a thin film of moisture in which they can live. Periodic dry spells provide a selective advantage to algae that can survive water stress. Chlamydomonas is a simple, unicellular chlorophyte with a pear-shaped morphology and two opposing, anterior flagella that guide this protist toward light sensed by its eyespot. These chlorophyte species exhibit haploid gametes and spores, which are also found in other more complex species.
The chlorophyte Volvox is one of only a few examples of a colonial organism, which behaves in some ways like a collection of individual cells, but in other ways like the specialized cells of a multicellular organism. Volvox colonies contain 500 to 60,000 cells, each with two flagella, contained within a hollow, spherical matrix composed of a gelatinous glycoprotein secretion. Individual Volvox cells move in a coordinated fashion and are interconnected by cytoplasmic bridges. Only a few of the cells reproduce to create daughter colonies, an example of basic cell specialization in this organism.
True multicellular organisms, such as the sea lettuce, Ulva, are also represented among the chlorophytes. Other chlorophytes exist as large, multinucleate, single cells. Within the genus Caulerpa some species undergo nuclear division, but their cells do not complete cytokinesis, remaining instead as massive and elaborate single cells.
While Chlorophytes share some features with land plants, the Charophytes are the closest living relatives to land plants and resemble them in both morphology and reproductive strategies. Within the charophytes, the order Charales, and the coleochaetes (microscopic green algae that enclose their spores in sporopollenin) are considered the closest living relatives of land plants. Charales have been discovered in the fossil record as far back as 420 million years ago. The organisms within this group live in a range of freshwater habitats and vary in size from a few millimeters to a meter in length.
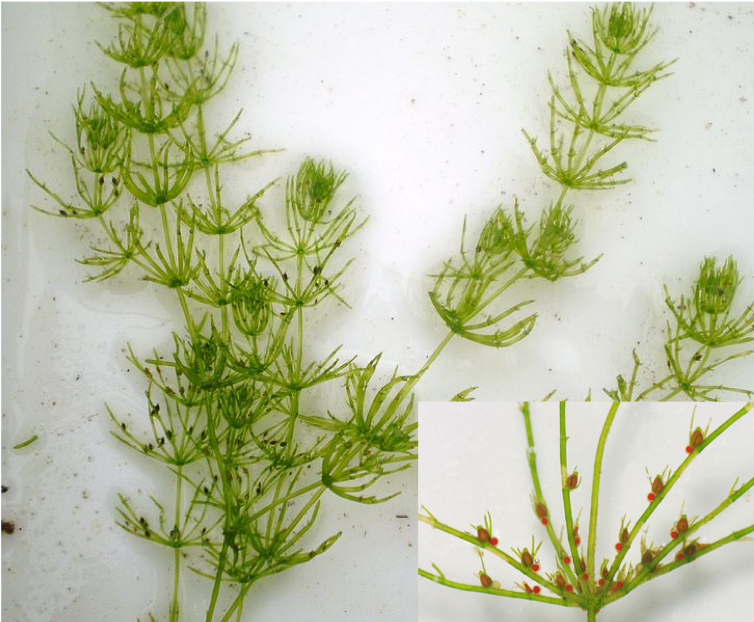
A representative species is Chara (Fig 3), which has large cells that form a thallus: the main stem of the alga. At the nodes, smaller cells can form branches and male and female reproductive structures are established. This group also produces flagellated sperm.
Unlike land plants, green algae within the Charales do not undergo alternation of generations in their lifecycle. However, Charales do exhibit a number of traits that are significant to the adaptation to land life. They produce the compound lignin, which provides support against gravity, and sporopollenin, which helps protect spores from drying out. In addition, they form plasmodesmata that connect the cytoplasm of adjacent cells which allows for greater ease in moving molecules including water between adjacent cells. Finally, the egg, and later, the zygote, form in a protected chamber on the parent plant, again this protects against drying out.
The separation of green algae from what are considered to be plants is one that is not always clear cut. While all algae are photosynthetic—that is, they contain some form of a chloroplast—they didn’t all become photosynthetic via the same path. In contrast, the evolutionary pathways of photosynthesis in land plants can be traced back to a common ancestor. The presence of cellulose in cell walls, chlorophyll a and later chlorophyll b as well as structural features of chloroplasts that provide the clearest connections between modern land plants and their algal ancestors.
Review Question:
Which of these DOES NOT provide evidence that green algae are the most likely ancestor of land plants?
A) the presence of chloroplasts
Summary
The Eukarya are a widely diverse group that is separated into six supergroups based on morphology and DNA evidence. Photosynthesis is found within four of those six groups, but only in the Archaeplastida are all members photosynthetic. In addition, many of the groups contain unicellular, simple multicellular and in some cases complex multicellular organisms. Land plants are considered to have evolved from green algal ancestors with the Charophytes due to a number of shared features including photosynthetic pigments, cellular structures, and reproductive strategies.
End of Section Review Questions:
A) some cell walls (chloroplast) that are composed of peptidoglycan
Review: Viridioplantae
Thinking Question
The chlorophyte (green algae) genera Ulva and Caulerpa both have macroscopic leaf-like and stem-like structures, but only Ulva species are considered truly multicellular. Explain why using your knowledge of multicellular features.
Learning Goals
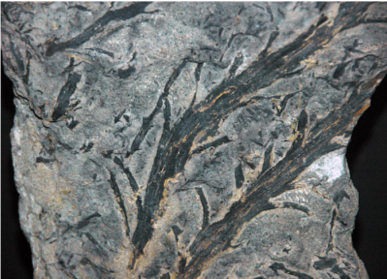
By the end of this reading you should be able to
- Discuss the challenges to plant life on land
- Describe common characteristics of all land plants
- Relate specific adaptive features to the unique challenges of life on land
Introduction
Current evolutionary thought holds that all plants—green algae as well as land dwellers—are monophyletic; that is, they are descendants of a single common ancestor. At some point in the evolutionary history of plants, there was a transition from an aquatic environment to the terrestrial one. This transition from water to land imposed severe constraints on plants. Plants had to develop strategies to avoid drying out, to disperse reproductive cells in air, to provide structural support, to exchange gases without water, and as they grew larger to move molecules by bulk flow. While some groups of plants developed adaptations that allowed them to populate even the aridest habitats on Earth, full independence from the water did not happen in all plants. As a group, most seedless plants still require a moist environment for at least some of their physiological functions. Land plants can be separated by the degree of development of their structures, both vascular as well as reproductive, and the variation in their alternation of generations lifecycle.
Plant Adaptations to Life on Land
As organisms adapted to life on land, they had to contend with several challenges in the terrestrial environment. Water has been described as “the stuff of life.” The cell’s interior is a watery soup: in this medium, most small molecules dissolve and diffuse, and the majority of the chemical reactions of metabolism take place. Desiccation, or drying out, is a constant danger for an organism exposed to air. Even when parts of a plant are close to a source of water, the aerial structures are likely to dry out. Water also provides buoyancy to organisms. On land, plants need to develop structural support in a medium that does not give the same lift as water. The organism is also subject to bombardment by mutagenic radiation because air does not filter out ultraviolet rays of sunlight. Additionally, the male gametes must reach the female gametes using new strategies, because swimming is no longer possible. Therefore, both gametes and zygotes must be protected from desiccation. The successful land plants developed strategies to deal with all of these challenges. Not all adaptations appeared at once. Some species never moved very far from the aquatic environment, whereas others went on to conquer the driest environments on Earth.
To balance these survival challenges, life on land offers several advantages. First, sunlight is abundant. Water acts as a filter, altering the spectral quality of light absorbed by the photosynthetic pigment chlorophyll. Second, carbon dioxide is more readily available in the air than in water, since it diffuses faster in air. Third, land plants evolved before land animals; therefore, until dry land was colonized by animals, no predators threatened plant life. This situation changed as animals emerged from the water and fed on the abundant sources of nutrients in the established flora. In turn, plants developed strategies that deterred predation: from spines and thorns to toxic chemicals.
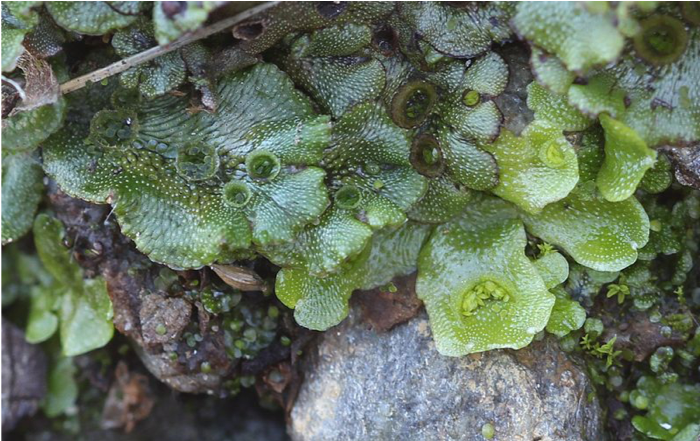
The most successful adaptation solution was the development of new structures that gave plants the advantage when colonizing new and dry environments. Four major adaptations are found in all terrestrial plants: the alternation of generations, a sporangium in which the spores are formed (Fig. 1), a gametangium that produces haploid cells, and apical meristem tissue involved in growth. The evolution of a waxy cuticle (Fig. 2) and a cell wall with lignin also contributed to the success of land plants, although not all land plants have lignin. These adaptations are noticeably lacking in the closely related green algae, further differentiating these two lineages.
Review Question:
Alternation of Generations
Alternation of generations describes a life cycle in which an organism has both haploid and diploid multicellular stages (Fig 3).
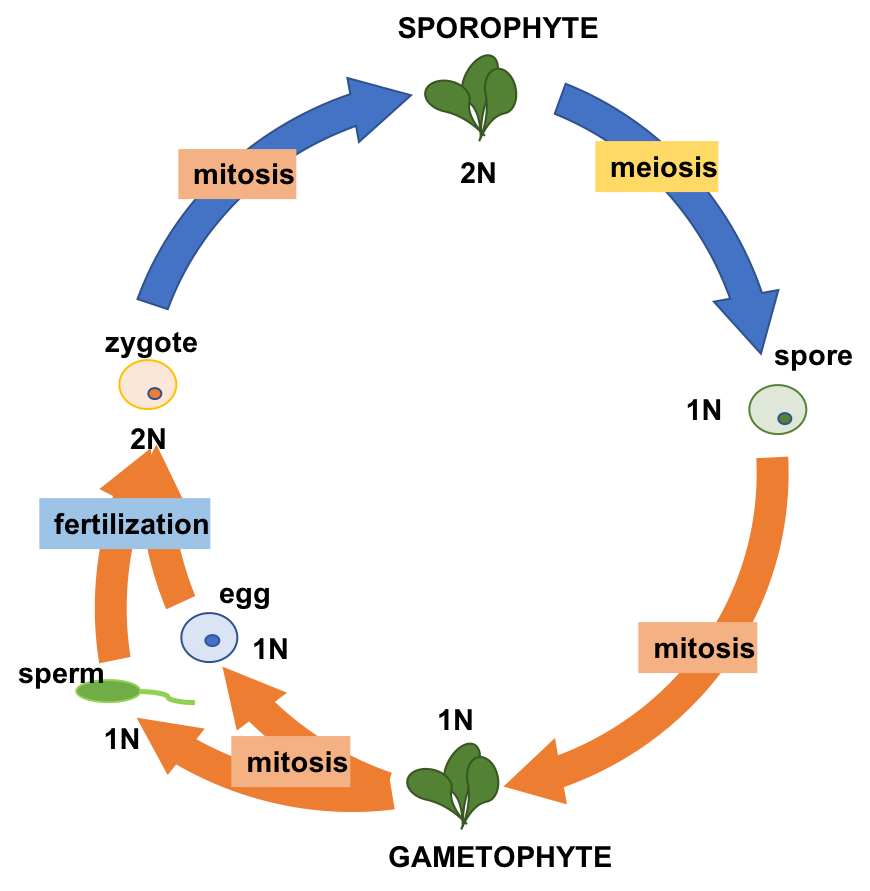
Regardless of the size of the gametophyte and/or sporophyte stage, the protection of the embryo is a major requirement for land plants. The vulnerable embryo must be sheltered from desiccation and other environmental hazards. In all land plants, the female gametophyte provides protection and nutrients to the embryo as it develops into the new generation of the sporophyte. This distinguishing feature of land plants, which is not present in green algae, makes all land plants embryophytes.
Review Question:
____________ occurs when gametophytes produce gametes
The zygote undergoes ____________ to become the multicellular ____________
Sporangia in Seedless Plants
The sporophyte of seedless plants is diploid and results from syngamy (fusion) of two gametes. The sporophyte bears the sporangia (singular, sporangium) (Fig. 4): organs that first appeared in the land plants. The term “sporangia” literally means “spore in a vessel,” as it is a reproductive sac that contains spores.
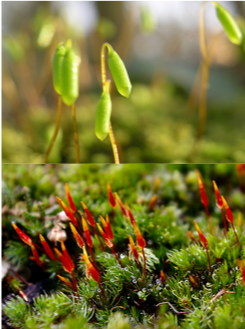
Inside the multicellular sporangia, the diploid sporocytes, or mother cells, produce haploid spores by meiosis. The spores are later released by the sporangia and disperse in the environment. Seedless non-vascular plants produce only one kind of spore and are called homosporous. The gametophyte phase is dominant in these plants. After germinating from a spore, the resulting gametophyte produces both male and female gametangia, usually on the same individual. In contrast, heterosporous plants produce two morphologically different types of spores (Fig. 1). The male spores are called microspores, because of their smaller size, and develop into the male gametophyte; the comparatively larger megaspores develop into the female gametophyte. Heterospory is observed in a few seedless vascular plants and in all seed plants.
The spores of seedless plants are surrounded by thick cell walls containing a tough polymer known as sporopollenin. This complex substance is characterized by long chains of organic molecules related to fatty acids and carotenoids: hence the yellow color of most pollen. Sporopollenin is unusually resistant to chemical and biological degradation. Sporopollenin was once thought to be an innovation of land plants; however, the green algae Coleochaetes forms spores that contain sporopollenin.
Gametangia in Seedless Plants
Gametangia (singular, gametangium) are structures observed on multicellular haploid gametophytes. In the gametangia, germline cells give rise to gametes by mitosis. The male gametangium (antheridium) releases sperm. Many seedless plants produce sperm equipped with flagella that enable them to swim in a moist environment to the archegonia: the female gametangium. The embryo develops inside the archegonium as the sporophyte. Gametangia are prominent in seedless plants but are very rarely found in seed plants.
Other adaptive features
- As plants adapted to dry land and became independent from the constant presence of water in damp habitats, new organs and structures made their appearance.
- Early land plants did not grow more than a few inches off the ground, competing for light on these low mats. By developing a shoot and growing taller, individual plants captured more light.
- Because air offers substantially less support than water, land plants incorporated more rigid molecules in their stems (and later, tree trunks).
- In small plants such as single-celled algae, simple diffusion suffices to distribute water and nutrients throughout the organism. However, for plants to evolve larger forms, the evolution of vascular tissue for the distribution of water and solutes was a prerequisite. The vascular system contains xylem and phloem tissues. Xylem conducts water and minerals absorbed from the soil up to the shoot, while phloem transports food derived from photosynthesis throughout the entire plant.
- A root system evolved to take up water and minerals from the soil and to anchor the increasingly taller shoot in the soil.
- In land plants, a waxy, waterproof cover called a cuticle protects the leaves and stems from desiccation.
- However, the cuticle also prevents the intake of carbon dioxide needed for the synthesis of carbohydrates through photosynthesis. To overcome this, stomata or pores that open and close to regulate the traffic of gases and water vapor appeared in plants as they moved away from moist environments into drier habitats.
- Water filters ultraviolet-B (UVB) light, which is harmful to all organisms, especially those that must absorb light to survive. This filtering does not occur for land plants. This presented an additional challenge to land colonization, which was met by the evolution of biosynthetic pathways for the synthesis of protective flavonoids and other compounds: pigments that absorb UV wavelengths of light and protect the aerial parts of plants from photodynamic damage.
- Plants cannot avoid being eaten by animals. Instead, they synthesize a large range of poisonous secondary metabolites: complex organic molecules such as alkaloids, whose noxious smells and unpleasant taste deter animals. These toxic compounds can also cause severe diseases and even death, thus discouraging predation. In contrast, as plants co-evolved with animals, the development of sweet and nutritious metabolites lured animals into providing valuable assistance in dispersing pollen grains, fruit, or seeds. Plants have been enlisting animals to be their helpers in this way for hundreds of millions of years.
Thinking Question:
Which trait(s) are likely to have evolved first as the ancestors of land plants adapted to more terrestrial environments? Defend your answer.
Summary
Land plants acquired traits that made it possible to colonize land and survive out of the water. All land plants share the following characteristics: alternation of generations, with the haploid plant called a gametophyte, and the diploid plant called a sporophyte; protection of the embryo, formation of haploid spores in a sporangium, the formation of gametes in a gametangium, and an apical meristem. Vascular tissues, roots, leaves, cuticle cover, and a tough outer layer that protects the spores contributed to the adaptation of plants to dry land.
End of Section Review Questions:
Review: Reproductive terminology
1) Match the term with the best description
1) Homosporous | A) undergo meiosis to produce haploid spores |
2) Microspore | B) producing two different types of spores |
3) Sporopollenin | C) polymer found in spore cell walls |
4) Sporocytes | D) produce only one type of spore (seedless non-vascular plants) |
5) Heterosporous | E) where precursor cells give rise to gametes by mitosis |
6) Gametangia | F) develops into the male gametophyte |
Review: Alternation of Generations
3) Which is true about alternation of generations in land plants? (Multiple Answers)
Image Attributions
Figure 1. Image courtesy of James St. John [CC BY 2.0 (https://creativecommons.org/licenses/by/2.0)]
Figure 2. Image courtesy of Avenue [GFDL (http://www.gnu.org/copyleft/fdl.html)]
Figure 3. Image created and provided by D. Jennings
Figure 4. Top Image courtesy of Guido Gerding - Own photograph, URL: Ex :: Natura - Freies Portal für Umweltbildung (Environmental Education), CC BY-SA 3.0, https://commons.wikimedia.org/w/index.php?curid=499406. Bottom Image courtesy of Vaelta at English Wikipedia. [CC BY-SA 3.0 (http://creativecommons.org/licenses/by-sa/3.0/)]
Learning Goals
- Describe fungal parasites and pathogens of plants
- Describe the different types of fungal infections in humans
- Explain why antifungal therapy is hampered by the similarity between fungal and animal cells
Parasitism describes a symbiotic relationship in which one member of the association benefits at the expense of the other. Both parasites and pathogens harm the host; however, the pathogen causes disease, whereas the parasite usually does not. Commensalism occurs when one member benefits without affecting the other.
Plant Parasites and Pathogens
The production of sufficient good-quality crops is essential to human existence. Plant diseases have ruined crops, bringing widespread famine. Many plant pathogens are fungi that cause tissue decay and eventual death of the host (Fig. 1). In addition to destroying plant tissue directly, some plant pathogens spoil crops by producing potent toxins. Fungi are also responsible for food spoilage and the rotting of stored crops. For example, the fungus Claviceps purpurea causes ergot, a disease of cereal crops (especially of rye). Although the fungus reduces the yield of cereals, the effects of the ergot's alkaloid toxins on humans and animals are of much greater significance. In animals, the disease is referred to as ergotism. The most common signs and symptoms are convulsions, hallucinations, gangrene, and loss of milk in cattle. The active ingredient of ergot is lysergic acid, which is a precursor of the drug LSD. Smuts, rusts, and powdery or downy mildew are other examples of common fungal pathogens that affect crops.

Aflatoxins are toxic, carcinogenic compounds released by fungi of the genus Aspergillus. Periodically, harvests of nuts and grains are tainted by aflatoxins, leading to a massive recall of produce. This sometimes ruins producers and causes food shortages in developing countries.
Animal and Human Parasites and Pathogens
Fungi can affect animals, including humans, in several ways. A mycosis is a fungal disease that results from infection and direct damage. Fungi attack animals directly by colonizing and destroying tissues. Mycotoxicosis is the poisoning of humans (and other animals) by foods contaminated by fungal toxins (mycotoxins). Mycetismus describes the ingestion of preformed toxins in poisonous mushrooms. In addition, individuals who display hypersensitivity to molds and spores develop strong and dangerous allergic reactions. Fungal infections are generally very difficult to treat because, unlike bacteria, fungi are eukaryotes. Antibiotics only target prokaryotic cells, whereas compounds that kill fungi also harm the eukaryotic animal host.
Many fungal infections are superficial; that is, they occur on the animal’s skin. Termed cutaneous (“skin”) mycoses, can have devastating effects. For example, the decline of the world’s frog population in recent years may be caused by the chytrid fungus Batrachochytrium dendrobatidis, which infects the skin of frogs and presumably interferes with gaseous exchange. Similarly, more than a million bats in the United States have been killed by white-nose syndrome, which appears as a white ring around the mouth of the bat. It is caused by the cold-loving fungus Geomyces destructans, which disseminates its deadly spores in caves where bats hibernate. Mycologists are researching the transmission, mechanism, and control of G. destructans to stop its spread.
Fungi that cause the superficial mycoses of the epidermis, hair, and nails rarely spread to the underlying tissue (Fig. 2). These fungi are often misnamed “dermatophytes”, from the Greek words dermis meaning skin and phyte meaning plant, although they are not plants. Dermatophytes are also called “ringworms” because of the red ring they cause on skin. They secrete extracellular enzymes that break down keratin (a protein found in hair, skin, and nails), causing conditions such as athlete’s foot and jock itch. These conditions are usually treated with over-the-counter topical creams and powders, and are easily cleared. More persistent superficial mycoses may require prescription oral medications.

Systemic mycoses spread to internal organs, most commonly entering the body through the respiratory system. For example, coccidioidomycosis (valley fever) is commonly found in the southwestern United States, where the fungus resides in the dust. Once inhaled, the spores develop in the lungs and cause symptoms similar to those of tuberculosis. Histoplasmosis is caused by the dimorphic fungus Histoplasma capsulatum. It also causes pulmonary infections, and in rarer cases, swelling of the membranes of the brain and spinal cord. Treatment of these and many other fungal diseases requires the use of antifungal medications that have serious side effects.
Opportunistic mycoses are fungal infections that are either common in all environments or part of the normal biota. They mainly affect individuals who have a compromised immune system. Patients in the late stages of AIDS suffer from opportunistic mycoses that can be life-threatening. The yeast Candida sp., a common member of the natural biota, can grow unchecked and infect the vagina or mouth (oral thrush) if the pH of the surrounding environment, the person’s immune defenses, or the normal population of bacteria is altered.
Mycetismus can occur when poisonous mushrooms are eaten. It causes a number of human fatalities during the mushroom-picking season. Many edible fruiting bodies of fungi resemble highly poisonous relatives, and amateur mushroom hunters are cautioned to carefully inspect their harvest and avoid eating mushrooms of doubtful origin. The adage “there are bold mushroom pickers and old mushroom pickers, but are there no old, bold mushroom pickers” is unfortunately true.
Dutch Elm Disease
Question: Do trees resistant to Dutch elm disease secrete antifungal compounds?
Hypothesis: Construct a hypothesis that addresses this question.
Background: Dutch elm disease is a fungal infestation that affects many species of elm (Ulmus) in North America. The fungus infects the vascular system of the tree, which blocks water flow within the plant and mimics drought stress. Accidentally introduced to the United States in the early 1930s, it decimated shade trees across the continent. It is caused by the fungus Ophiostoma ulmi. The elm bark beetle acts as a vector and transmits the disease from tree to tree. Many European and Asiatic elms are less susceptible to the disease than are American elms.
Explain how you would go about testing your hypothesis for this theoretical Dutch Elm experiment. Include independent and dependent variables, your controls, what you will measure, and how you will analyze your data.
SUMMARY
Fungi establish parasitic relationships with plants and animals. Fungal diseases can decimate crops and spoil food during storage. Compounds produced by fungi can be toxic to humans and other animals. Mycoses are infections caused by fungi. Superficial mycoses affect the skin, whereas systemic mycoses spread through the body. Fungal infections are difficult to cure.
End of Section Review Questions:
Review: Infections
Review: Human diseasesWhy can superficial mycoses in humans lead to bacterial infections?
Learning Goals
- Describe the importance of fungi to the balance of the environment
- Summarize the role of fungi in food and beverage preparation
- Describe the importance of fungi in the chemical and pharmaceutical industries
- Discuss the role of fungi as model organisms
Although we often think of fungi as organisms that cause disease and rot food, fungi are important to human life on many levels. As we have seen, they influence the well-being of human populations on a large scale because they are part of the nutrient cycle in ecosystems. They have other ecosystem roles as well. As animal pathogens, fungi help to control the population of damaging pests. These fungi are very specific to the insects they attack and do not infect animals or plants. Fungi are currently under investigation as potential microbial insecticides, with several already on the market. For example, the fungus Beauveria bassiana is a pesticide being tested as a possible biological control agent for the recent spread of emerald ash borer. It has been released in Michigan, Illinois, Indiana, Ohio, West Virginia, and Maryland (Fig. 1).

The mycorrhizal relationship between fungi and plant roots is essential for the productivity of farmland. Without the fungal partner in root systems, 80–90 percent of trees and grasses would not survive. Mycorrhizal fungal inoculants are available as soil amendments from gardening supply stores and are promoted by supporters of organic agriculture.
We also eat some types of fungi. Mushrooms figure prominently in the human diet. Morels, shiitake mushrooms, chanterelles, and truffles are considered delicacies (Fig. 2). The humble meadow mushroom, Agaricus campestris, appears in many dishes. Molds of the genus Penicillium ripen many kinds of cheese. They originate in the natural environment such as the caves of Roquefort, France, where wheels of sheep milk cheese are stacked in order to capture the molds responsible for the blue veins and pungent taste of the cheese.

Fermentation—of grains to produce beer, and of fruits to produce wine—is an ancient art that humans in most cultures have practiced for millennia. Wild yeasts are acquired from the environment and used to ferment sugars into CO2 and ethyl alcohol under anaerobic conditions. It is now possible to purchase isolated strains of wild yeasts from different wine-making regions. Louis Pasteur was instrumental in developing a reliable strain of brewer’s yeast, Saccharomyces cerevisiae, for the French brewing industry in the late 1850s. This was one of the first examples of biotechnology patenting.
Many secondary metabolites of fungi are of great commercial importance. Antibiotics are naturally produced by fungi to kill or inhibit the growth of bacteria, limiting their competition in the natural environment. Important antibiotics, such as penicillin and the cephalosporins, are isolated from fungi. Valuable drugs isolated from fungi include the immunosuppressant drug cyclosporine (which reduces the risk of rejection after an organ transplant), the precursors of steroid hormones, and ergot alkaloids used to stop bleeding. Psilocybin is a compound found in fungi such as Psilocybe semilanceata and Gymnopilus junonius, which have been used for their hallucinogenic properties by various cultures for thousands of years.
As simple eukaryotic organisms, fungi are important model research organisms. Many advances in modern genetics were achieved by the use of the red bread mold Neurospora crassa. Additionally, many important genes originally discovered in S. cerevisiae served as a starting point in discovering analogous human genes. As a eukaryotic organism, the yeast cell produces and modifies proteins in a manner similar to human cells, as opposed to the bacterium Escherichia coli, which lacks the internal membrane structures and enzymes to tag proteins for export. This makes yeast a much better organism for use in recombinant DNA technology experiments. Like bacteria, yeasts grow easily in culture, have a short generation time, and are amenable to genetic modification.
SUMMARY
Fungi are important to everyday human life. Fungi are important decomposers in most ecosystems. Mycorrhizal fungi are essential for the growth of most plants. Fungi, as food, play a role in human nutrition in the form of mushrooms, and also as agents of fermentation in the production of bread, cheeses, alcoholic beverages, and numerous other food preparations. Secondary metabolites of fungi are used as medicines, such as antibiotics and anticoagulants. Fungi are model organisms for the study of eukaryotic genetics and metabolism.
Review: Wine and Beer!
1) Yeast is a facultative anaerobe. This means that alcohol fermentation takes place only if:
Review: Biotechnology
2) The advantage of yeast cells over bacterial cells to express human proteins is that:
Review: mmmmmm... bread...
3) Historically, artisanal breads were produced by capturing wild yeasts from the air. Prior to the development of modern yeast strains, the production of artisanal breads was long and laborious because many batches of dough ended up being discarded. Can you explain this fact?
Learning Goals
- Describe the importance of fungi to the balance of the environment
- Summarize the role of fungi in food and beverage preparation
- Describe the importance of fungi in the chemical and pharmaceutical industries
- Discuss the role of fungi as model organisms
Although we often think of fungi as organisms that cause disease and rot food, fungi are important to human life on many levels. As we have seen, they influence the well-being of human populations on a large scale because they are part of the nutrient cycle in ecosystems. They have other ecosystem roles as well. As animal pathogens, fungi help to control the population of damaging pests. These fungi are very specific to the insects they attack and do not infect animals or plants. Fungi are currently under investigation as potential microbial insecticides, with several already on the market. For example, the fungus Beauveria bassiana is a pesticide being tested as a possible biological control agent for the recent spread of emerald ash borer. It has been released in Michigan, Illinois, Indiana, Ohio, West Virginia, and Maryland (Fig. 1).

The mycorrhizal relationship between fungi and plant roots is essential for the productivity of farmland. Without the fungal partner in root systems, 80–90 percent of trees and grasses would not survive. Mycorrhizal fungal inoculants are available as soil amendments from gardening supply stores and are promoted by supporters of organic agriculture.
We also eat some types of fungi. Mushrooms figure prominently in the human diet. Morels, shiitake mushrooms, chanterelles, and truffles are considered delicacies (Fig. 2). The humble meadow mushroom, Agaricus campestris, appears in many dishes. Molds of the genus Penicillium ripen many kinds of cheese. They originate in the natural environment such as the caves of Roquefort, France, where wheels of sheep milk cheese are stacked in order to capture the molds responsible for the blue veins and pungent taste of the cheese.

Fermentation—of grains to produce beer, and of fruits to produce wine—is an ancient art that humans in most cultures have practiced for millennia. Wild yeasts are acquired from the environment and used to ferment sugars into CO2 and ethyl alcohol under anaerobic conditions. It is now possible to purchase isolated strains of wild yeasts from different wine-making regions. Louis Pasteur was instrumental in developing a reliable strain of brewer’s yeast, Saccharomyces cerevisiae, for the French brewing industry in the late 1850s. This was one of the first examples of biotechnology patenting.
Many secondary metabolites of fungi are of great commercial importance. Antibiotics are naturally produced by fungi to kill or inhibit the growth of bacteria, limiting their competition in the natural environment. Important antibiotics, such as penicillin and the cephalosporins, are isolated from fungi. Valuable drugs isolated from fungi include the immunosuppressant drug cyclosporine (which reduces the risk of rejection after an organ transplant), the precursors of steroid hormones, and ergot alkaloids used to stop bleeding. Psilocybin is a compound found in fungi such as Psilocybe semilanceata and Gymnopilus junonius, which have been used for their hallucinogenic properties by various cultures for thousands of years.
As simple eukaryotic organisms, fungi are important model research organisms. Many advances in modern genetics were achieved by the use of the red bread mold Neurospora crassa. Additionally, many important genes originally discovered in S. cerevisiae served as a starting point in discovering analogous human genes. As a eukaryotic organism, the yeast cell produces and modifies proteins in a manner similar to human cells, as opposed to the bacterium Escherichia coli, which lacks the internal membrane structures and enzymes to tag proteins for export. This makes yeast a much better organism for use in recombinant DNA technology experiments. Like bacteria, yeasts grow easily in culture, have a short generation time, and are amenable to genetic modification.
SUMMARY
Fungi are important to everyday human life. Fungi are important decomposers in most ecosystems. Mycorrhizal fungi are essential for the growth of most plants. Fungi, as food, play a role in human nutrition in the form of mushrooms, and also as agents of fermentation in the production of bread, cheeses, alcoholic beverages, and numerous other food preparations. Secondary metabolites of fungi are used as medicines, such as antibiotics and anticoagulants. Fungi are model organisms for the study of eukaryotic genetics and metabolism.
Review: Wine and Beer!
1) Yeast is a facultative anaerobe. This means that alcohol fermentation takes place only if:
Review: Biotechnology
2) The advantage of yeast cells over bacterial cells to express human proteins is that:
Review: mmmmmm... bread...
3) Historically, artisanal breads were produced by capturing wild yeasts from the air. Prior to the development of modern yeast strains, the production of artisanal breads was long and laborious because many batches of dough ended up being discarded. Can you explain this fact?
Learning Goals
By the end of this reading you should be able to:
- Identify the two supergroups of Eukarya that contain plants, animals, and fungi
- Explain why the presence of photosynthesis is not used as a defining characteristic of Eukaryotic supergroups
- Compare the traits shared by green algae and land plants
- Explain the reasons why Charales are considered the closest relative to land plants
Introduction
The oldest fossils appear to all be prokaryotes, while the evidence of eukaryotes is about 2 billion years old (they appear later in the fossil record). Based on this it is probable that today’s eukaryotes are descended from an ancestor that had a prokaryotic organization. The last common ancestor of today’s Eukarya had several characteristics, including cells with nuclei that divided mitotically and contained linear chromosomes where the DNA was associated with histones, a cytoskeleton and endomembrane system, and the ability to make cilia/flagella during at least part of its life cycle. This ancestor was aerobic because it had mitochondria that were the result of endosymbiosis of an aerobic alpha-proteobacterium. Whether this ancestral cell had a nucleus at the time of the initial symbiosis remains unknown. Modern Eukarya are an amazingly diverse group of organisms, from single cells to complex multicellular organisms and with multiple means of acquiring both energy and the carbon need for building blocks.
Supergroups within the Eukarya
The emerging classification scheme groups the entire domain Eukaryota into six “supergroups” that evolved from a common ancestor and contains all of the protists as well as animals, plants, and fungi (Fig. 1).
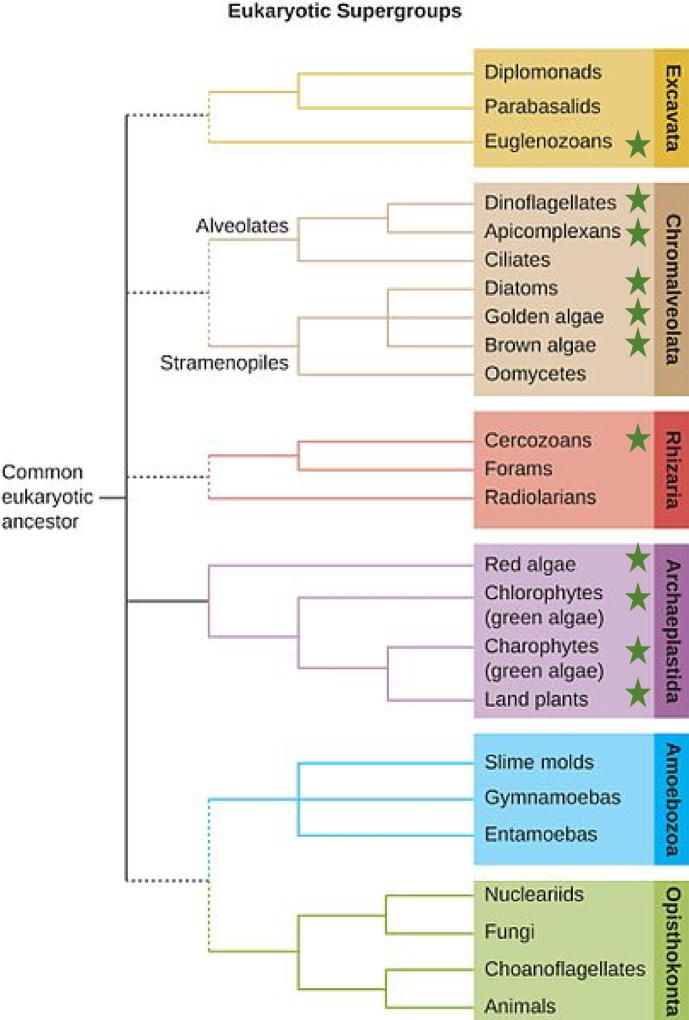
The supergroups are believed to be monophyletic, meaning that all organisms within each supergroup are believed to have evolved from a single common ancestor, and thus all members are most closely related to each other than to organisms outside that group. There is still evidence lacking for the monophyly of some groups.
The classification of eukaryotes is still in flux, and the six supergroups may be modified or replaced by a more appropriate hierarchy as genetic, morphological, and ecological data accumulate. Keep in mind that the classification scheme presented here is just one of several hypotheses, and the true evolutionary relationships are still to be determined.
Archaeplastida
The supergroup Archaeplastida is separated into three phylogenetic groups: the Glaucocytophyes, the Red Algae, and the Viridioplantae. With only a few exceptions all of the organisms within the supergroup are photosynthetic. Photosynthesis, however, is found in other groups that are not within the Archaeplastida, including the brown and golden algae, diatoms, dinoflagellates, some apicomplexans, some excavata (Euglenids), and some Rhizarians (see Fig. 2). Red algae and green algae, however, are included in the supergroup Archaeplastida. Molecular evidence supports that all Archaeplastida are descendants of an endosymbiotic relationship between a heterotrophic protist and a cyanobacterium.
Thinking Question:
What is a possible explanation for the pattern of distribution of photosynthetic eukaryotes (they are widely distributed but discontinuously among the different groups)? Be sure to provide support for your reasoning.
Glaucophytes:
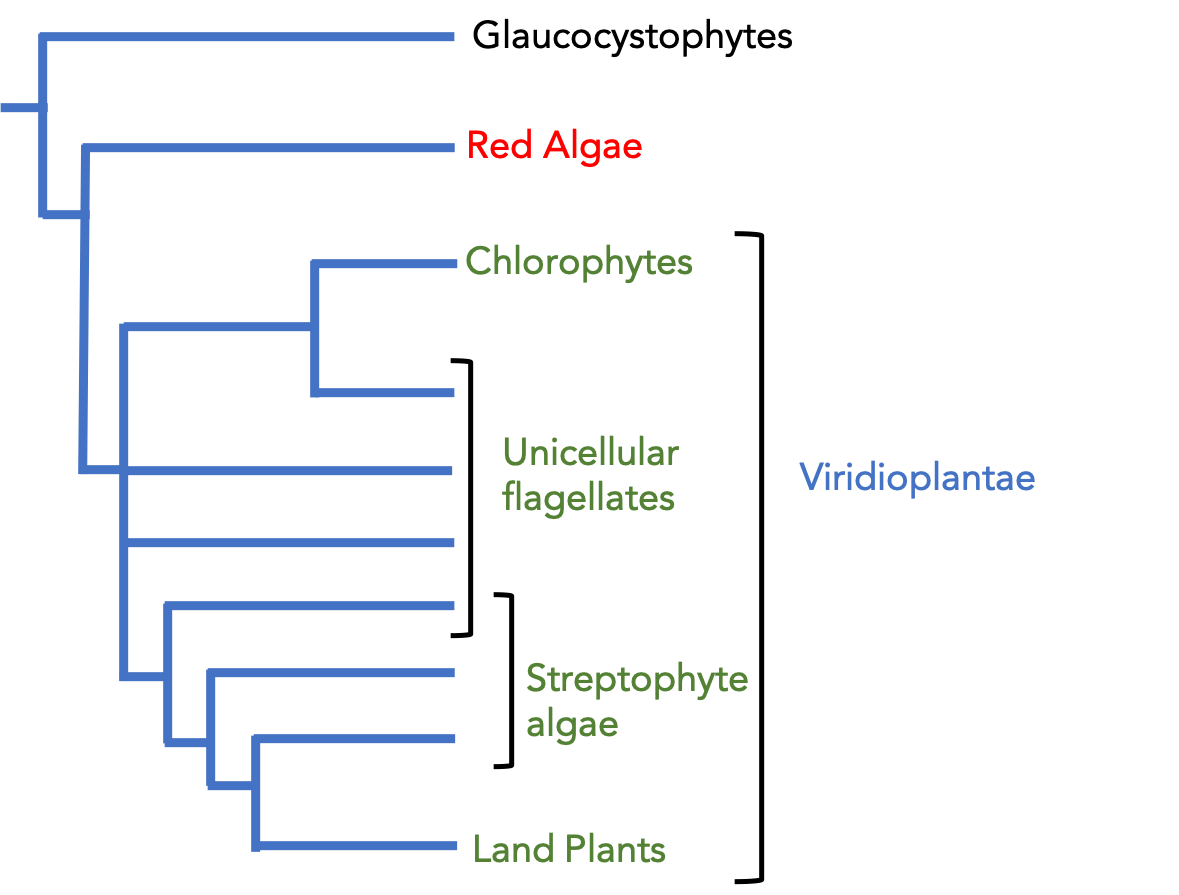
This is a small group of organisms that reside in freshwater ponds and lakes. They are unique in that their chloroplasts have walls of peptidoglycan, a feature retained from the ancestral cyanobacterial endosymbiont. In addition, their photosynthetic pigments include biliprotein which is also found in cyanobacteria.
Red Algae
Red algae, or rhodophytes, are primarily multicellular, most lack flagella, and range in size from microscopic, unicellular protists to large, multicellular forms that have differentiated tissues and in some cases complex morphology. Red algae, like land plants, have cell walls composed of cellulose and display a life cycle with an alternation of generations. There are many species of red algae that are seaweeds that are found in the shallow seafloor, however, and some species have unique pigment photosynthetic accessory pigments. These phycoerythrin pigments are red in color which allows the algae to utilize short (blue) wavelengths of light. These wavelengths are found at greater depths than the red wavelengths commonly used by other photosynthetic organisms and thus allow these algae to live at greater depths and outcompete other algae. Coralline red algae are found at the edges of coral reefs and they have the ability to secrete calcium carbonate (CaCO3) to form an external skeleton-like structure that protects them from the motion of the waves. These tiny red algae are key to the establishment of the coral reefs as they provide a needed buffer for the newly attached corals.
Viridioplantae: Green Algae
Green algae are the most abundant group of algae and exhibit similar features to the land plants. The cells in green algae divide along cell plates called phragmoplasts, and their cell walls are layered in the same manner as the cell walls of land plants. In addition, green algae contain the same carotenoids and chlorophyll a and b as land plants, whereas other algae have different accessory pigments and types of chlorophyll molecules in addition to chlorophyll a. Both green algae and land plants also store carbohydrates like starch. These similarities provide evidence that, even though green algae are classified as protists, they shared a relatively recent common ancestor with land plants.
There is wide diversity within the green algae, and while some groups are unicellular and flagellate, the Chlorophytes and the Charophytes contain members that are complex and multicellular. The Chlorophytes include more than 7000 different species that live in fresh or brackish water, in seawater, or in snow patches. A few even survive on soil, provided it is covered by a thin film of moisture in which they can live. Periodic dry spells provide a selective advantage to algae that can survive water stress. Chlamydomonas is a simple, unicellular chlorophyte with a pear-shaped morphology and two opposing, anterior flagella that guide this protist toward light sensed by its eyespot. These chlorophyte species exhibit haploid gametes and spores, which are also found in other more complex species.
The chlorophyte Volvox is one of only a few examples of a colonial organism, which behaves in some ways like a collection of individual cells, but in other ways like the specialized cells of a multicellular organism. Volvox colonies contain 500 to 60,000 cells, each with two flagella, contained within a hollow, spherical matrix composed of a gelatinous glycoprotein secretion. Individual Volvox cells move in a coordinated fashion and are interconnected by cytoplasmic bridges. Only a few of the cells reproduce to create daughter colonies, an example of basic cell specialization in this organism.
True multicellular organisms, such as the sea lettuce, Ulva, are also represented among the chlorophytes. Other chlorophytes exist as large, multinucleate, single cells. Within the genus Caulerpa some species undergo nuclear division, but their cells do not complete cytokinesis, remaining instead as massive and elaborate single cells.
While Chlorophytes share some features with land plants, the Charophytes are the closest living relatives to land plants and resemble them in both morphology and reproductive strategies. Within the charophytes, the order Charales, and the coleochaetes (microscopic green algae that enclose their spores in sporopollenin) are considered the closest living relatives of land plants. Charales have been discovered in the fossil record as far back as 420 million years ago. The organisms within this group live in a range of freshwater habitats and vary in size from a few millimeters to a meter in length.
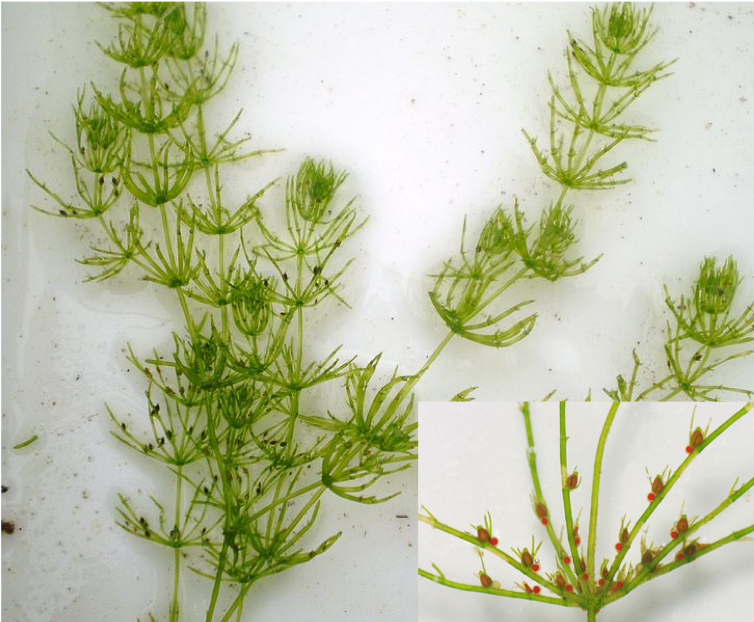
A representative species is Chara (Fig 3), which has large cells that form a thallus: the main stem of the alga. At the nodes, smaller cells can form branches and male and female reproductive structures are established. This group also produces flagellated sperm.
Unlike land plants, green algae within the Charales do not undergo alternation of generations in their lifecycle. However, Charales do exhibit a number of traits that are significant to the adaptation to land life. They produce the compound lignin, which provides support against gravity, and sporopollenin, which helps protect spores from drying out. In addition, they form plasmodesmata that connect the cytoplasm of adjacent cells which allows for greater ease in moving molecules including water between adjacent cells. Finally, the egg, and later, the zygote, form in a protected chamber on the parent plant, again this protects against drying out.
The separation of green algae from what are considered to be plants is one that is not always clear cut. While all algae are photosynthetic—that is, they contain some form of a chloroplast—they didn’t all become photosynthetic via the same path. In contrast, the evolutionary pathways of photosynthesis in land plants can be traced back to a common ancestor. The presence of cellulose in cell walls, chlorophyll a and later chlorophyll b as well as structural features of chloroplasts that provide the clearest connections between modern land plants and their algal ancestors.
Review Question:
Which of these DOES NOT provide evidence that green algae are the most likely ancestor of land plants?
A) the presence of chloroplasts
Summary
The Eukarya are a widely diverse group that is separated into six supergroups based on morphology and DNA evidence. Photosynthesis is found within four of those six groups, but only in the Archaeplastida are all members photosynthetic. In addition, many of the groups contain unicellular, simple multicellular and in some cases complex multicellular organisms. Land plants are considered to have evolved from green algal ancestors with the Charophytes due to a number of shared features including photosynthetic pigments, cellular structures, and reproductive strategies.
End of Section Review Questions:
A) some cell walls (chloroplast) that are composed of peptidoglycan
Review: Viridioplantae
Thinking Question
The chlorophyte (green algae) genera Ulva and Caulerpa both have macroscopic leaf-like and stem-like structures, but only Ulva species are considered truly multicellular. Explain why using your knowledge of multicellular features.