
Scientists seek to understand the fundamental principles that explain natural patterns and processes. Science is more than just a body of knowledge, science provides a means to evaluate and create new knowledge while minimizing bias [1].
Scientists use objective evidence over subjective evidence, to reach sound and logical conclusions. An objective observation is without personal bias and the same by all individuals. Humans are biased by nature, so they cannot be completely objective; the goal is to be as unbiased as possible. A subjective observation is based on a person’s feelings and beliefs and is unique to that individual.
Another way scientists avoid bias is by using quantitative over qualitative measurements whenever possible. A quantitative measurement is expressed with a specific numerical value. Qualitative observations are general or relative descriptions. For example, describing a tree as tall or young is a qualitative observation. Measuring the height of the tree and using tree ring data to determine the tree age is quantitative. Numerical values are more precise than general descriptions, and they can be analyzed using statistical calculations. This is why quantitative measurements are much more useful to scientists than qualitative observations.
Falsifiability separates science from pseudoscience. Scientists are wary of explanations of natural phenomena that discourage or avoid falsifiability. An explanation that cannot be tested or does not meet scientific standards is not considered science, but pseudoscience. Pseudoscience is a collection of ideas that may appear scientific but does not use the scientific method. Astrology is an example of pseudoscience. It is a belief system that claims that the movement of celestial bodies influences human behavior. Astrologers rely on celestial observations, but their conclusions are not based on experimental evidence and their statements are not falsifiable. This is not to be confused with astronomy which is the scientific study of celestial bodies and the cosmos [2; 3]. Establishing truth in science is difficult because all scientific claims are falsifiable, which means any initial hypothesis may be tested and proven false. A hypothesis a specific, testable explanation of how multiple variables and observations relate (for example, increased nitrogen in the soil will have increase the fruit production of tomatoes more than it will increase the fruit production of snap peas when fruit production is measured as the total mass of fruit). One common misconception is that if a hypothesis is supported, it becomes a theory. This is used to help distinguish between the typical use of “theory” in everyday conversation and overarching theories in science like gravity and evolution. A scientific theory is a broad explanation for how something in the world works based on observations and previous research. A hypothesis is much more specific. If repeated testing of hypotheses generated from the theory indicates that the theory is a good explanation for the phenomena, the theory gains acceptance, but the hypotheses do not become theories. Similarly, lots of hypotheses that have been confirmed can be pulled together to form a theory. A theory is always subject to revision or even rejection based on new evidence and testing of hypotheses generated from the theory or from competing theories. This meticulous scrutiny reveals weaknesses or flaws in a hypothesis and is the strength that supports all scientific ideas and procedures. In fact, proving current ideas are wrong has been the driving force behind many scientific careers. Despite this, some theories explain such a wide range of phenomena and are so well-supported, that they can be considered overarching or fundamental theories. Examples include plate tectonics, evolution, and special relativity.
1.2 The Scientific Method
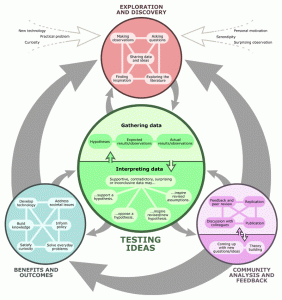
Modern science is based on the scientific method, an iterative process in which scientists
- Formulate a question or observe a problem (Exploration and discovery)
- Apply objective experimentation and observation (Gathering data)
- Analyze collected data and interpret results (Interpreting data)
- Submit findings to peer review and publication (Community analysis and feedback)
This has a long history in human thought but was first fully formed by Ibn al-Haytham over 1,000 years ago. At the forefront of the scientific method are conclusions based on objective evidence, not opinion or hearsay [4]. The scientific methods is also not a linear path. Students often think that the purpose of scientific exploration is to “prove” a hypothesis. In fact, the goal of science is always to “test” the hypothesis and then to share the findings with the larger scientific community to help build our collective understanding of the world.
STEP ONE: FORMULATE A QUESTION OR OBSERVE A PROBLEM
The procedure begins with identifying a problem or research question, such as a phenomenon that is not well explained in the scientific community’s collective knowledge. This step usually involves reviewing the scientific literature to understand previous studies that may be related to the question. This step can also be one of the most creative steps in the scientific method. Consider Alexander Fleming’s discovery of penicillin. The story is often told that Fleming discovered penicillin by accident, which is partially true. Fleming was studying staphylococcal bacteria and left a petri dish sitting out while on vacation (some say it was supposed to be in the incubator, some reports say it was supposed to be thrown away). When he returned, he found that there were areas on the petri dish where the bacteria was dying. This was a chance observation, but Fleming’s previous work on antimicrobial substances helped him understand the observation and develop and complete testing that led to the discovery of penicillin. The story of Alexander Fleming illustrates how knowledge of the field, previous research, chance, careful observation, and some creativity can all come together to help develop a research question and an approach to answering that question.
Once the problem or question is well defined, the scientist proposes a possible answer, a hypothesis, before conducting an experiment or fieldwork. This hypothesis must be specific, falsifiable, and should be based on other scientific work. Most people have been taught at some point that a hypothesis is an “educated guess.” This definition has some merit, in that a good hypothesis is based on an understanding of similar phenomena, previous experience, and education, but a hypothesis must be testable. A scientist’s job is never to prove a hypothesis but to test the hypothesis.
STEP TWO: APPLY OBJECTIVE EXPERIMENTATION AND OBSERVATION
The next step is developing a way of testing the hypothesis. More accurately, scientists often test a prediction based on the hypothesis. Scientists often use controlled experiments at this step, but computer models, analysis of existing data sets, and long-term observations can also be used to test the hypothesis. For example, one of the most impactful research projects in environmental science is the long-term record of atmospheric CO2 concentrations from the Mauna Loa Observatory. The graph of this record, the Keeling Curve, demonstrated both that atmospheric CO2 varies with a predictable seasonal pattern and that atmospheric CO2 is increasing. Many people mistakenly think experiments are only done in a lab; however, an experiment can consist of observing natural processes in the field. In some cases, scientists use natural experiments, like the recovery of a forest after a fire, or compare different ecosystems, such as examining the animal species living in an agricultural field vs a neighboring meadow. Regardless of how a scientist tests a hypothesis, it always includes the systematic gathering of objective data.
STEP THREE: ANALYZE COLLECTED DATA AND INTERPRET RESULTS
The objective data is interpreted to determine whether it contradicts or supports the hypothesis. Data interpretation and analysis is a key step in the experimentation process. Scientists do not simply record their data, make a graph and finish. Scientists use their understanding of the field to determine what the data means. In scientific writing, scientists typically separate this interpretation step (the discussion) from the concrete data (the results) in the experiment. On occasion, scientists may need to revise their experimental approach. This is not done because the data is not supporting the hypothesis, but should only be done if the scientist discovers that the current experimental approach is not effective at testing the hypothesis and answering the research question.
STEP FOUR: SUBMIT FINDINGS TO PEER REVIEW AND PUBLICATION
Scientists share the results of their research by publishing articles in scientific journals, such as Science and Nature, and presenting at conferences. Reputable journals and publishing houses will not publish an experimental study until they have determined its methods are scientifically rigorous and the conclusions are supported by evidence. Before an article is published, it undergoes a rigorous peer review by scientific experts who scrutinize the methods, results, and discussion. Once an article is published, other scientists may attempt to replicate the results. This replication is necessary to confirm the reliability of the study’s reported results. A hypothesis that seemed compelling in one study might be proven false in studies conducted by other scientists. Scientific thinking requires that scientists revise their ideas in light of new evidence. New technology can be applied to published studies, which can aid in confirming or rejecting once-accepted ideas and/or hypotheses. Scientific discovery is an ongoing process and a key element is that scientists present their work for scrutiny by others and for others to use for their own research.
This process seems straightforward and linear, but in fact, scientists repeat steps, loopback in the process, and get stuck all the time. The TED talk below gives a more accurate description of how science is done.
Another key aspect of the scientific process is the impact and benefits of the research. Sometimes, research impacts policy or human behavior. Sometimes it leads to a new medication. Sometimes, it leads to more research which then has a broader impact. When Alexander Fleming discovered penicillin, his initial discovery did not have much impact. It was not until other scientists discovered how to produce penicillin and it was used to prevent infections in World War II that the impact and benefits of Fleming’s work were realized.
1.3 Basic and Applied Science
Is it valuable to pursue science for the sake of simply gaining knowledge, or does scientific knowledge only have worth if we can apply it to solving a specific problem or bettering our lives? This question focuses on the differences between two types of science: basic science and applied science.
Basic science or “pure” science seeks to expand knowledge regardless of the short-term application of that knowledge. It is not focused on developing a product or a service of immediate public or commercial value. The immediate goal of basic science is knowledge for knowledge’s sake, though this does not mean that in the end, it may not result in an application.
In contrast, applied science aims to use science to solve real-world problems, such as improving crop yield, find a cure for a particular disease, or save animals threatened by a natural disaster. In applied science, the problem is usually defined for the researcher and the researcher develops a way to solve the problem.
Some individuals may perceive applied science as “useful” and basic science as “useless.” A question these people might pose to a scientist advocating knowledge acquisition would be, “What for?” A careful look at the history of science, however, reveals that basic knowledge has resulted in many remarkable applications of great value. Many scientists think that a basic understanding of science is necessary before an application is developed; therefore, applied science relies on the results generated through basic science. Other scientists think that it is time to move on from basic science and instead to find solutions to actual problems. Both approaches are valid. It is true that there are problems that demand immediate attention; however, few solutions would be found without the help of the knowledge generated through basic science.
One example of how basic and applied science can work together to solve practical problems occurred after the discovery of DNA structure led to an understanding of the molecular mechanisms governing DNA replication. Strands of DNA, unique in every human, are found in our cells, where they provide the instructions necessary for life. During DNA replication, new copies of DNA are made, shortly before a cell divides to form new cells. Understanding the mechanisms of DNA replication (through basic science) enabled scientists to develop laboratory techniques that are now used to identify genetic diseases, pinpoint individuals who were at a crime scene, and determine paternity (all examples of applied science). Without basic science, it is unlikely that applied science would exist.
Another example of the link between basic and applied research is the Human Genome Project, a study in which each human chromosome was analyzed and mapped to determine the precise sequence of the DNA code and the exact location of each gene. (The gene is the basic unit of heredity; an individual’s complete collection of genes is his or her genome.) Other organisms have also been studied as part of this project to gain a better understanding of human chromosomes. The Human Genome Project (Figure 5) relied on basic research carried out with non-human organisms and, later, with the human genome. An important end goal eventually became using the data for applied research seeking cures for genetic diseases.
1.4 Science Denial
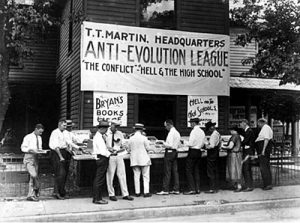
Introductory science courses usually deal with accepted scientific theory and do not include opposing ideas, even though these alternate ideas may be credible. This makes it easier for students to understand the complex material. Advanced students will encounter more controversies as they continue to study their discipline. Continually re-evaluating theories based on new evidence is a hallmark of science. However, some groups of people argue that some established scientific theories are wrong, not based on their scientific merit but rather on the ideology of the group. This section focuses on how to identify evidence-based information and differentiate it from pseudoscience.

Science denial happens when people argue that established scientific theories are wrong, not based on scientific merit but rather on subjective ideology—such as for social, political, or economic reasons. Organizations and people use science denial as a rhetorical argument against issues or ideas they oppose. Three examples of science denial versus science are: 1) teaching evolution in public schools, 2) linking tobacco smoke to cancer, and 3) linking human activity to climate change. Among these, denial of climate change is strongly connected with geology. A climate denier specifically denies or doubts the objective conclusions of geologists and climate scientists.
Science denial generally uses three false arguments. The first argument tries to undermine the credibility of the scientific conclusion by claiming the research methods are flawed or the theory is not universally accepted—the science is unsettled.
The notion that scientific ideas are not absolute creates doubt for non-scientists; however, a lack of universal truths should not be confused with scientific uncertainty. Because science is based on falsifiability, scientists avoid claiming universal truths and use language that conveys uncertainty. This allows scientific ideas to change and evolve as more evidence is uncovered. For example, climate deniers argue that scientists do not all agree that humans are the primary cause of climate change. While this is technically true, the overwhelming majority of climate scientists, those scientists with expertise in the field agree.
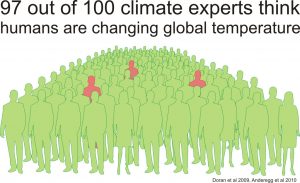

The second argument claims the researchers are not objective and motivated by an ideology or economic agenda. This is an ad hominem argument in which a person’s character is attacked instead of the merit of their argument. They claim results have been manipulated so researchers can justify asking for more funding. They claim that because the researchers are funded by a federal grant, they are using their results to lobby for expanded government regulation.
The third argument is to demand a balanced view, equal time in media coverage and educational curricula, to engender the false illusion of two equally valid arguments. Science deniers frequently demand equal coverage of their proposals, even when there is little scientific evidence supporting their ideology. For example, science deniers might demand religious explanations be taught as an alternative to the well-established theory of evolution [5; 6]. Or that all possible causes of climate change be discussed as equally probable, regardless of the body of evidence. Conclusions derived using the scientific method should not be confused with those based on ideologies.
Furthermore, conclusions about nature derived from ideologies have no place in science research and education. For example, it would be inappropriate to teach the flat earth model in a modern geology course because this idea has been disproved by the scientific method. Unfortunately, widespread scientific illiteracy allows these arguments to be used to suppress scientific knowledge and spread misinformation.
The formation of new conclusions based on the scientific method is the only way to change scientific conclusions. We wouldn’t teach Flat Earth geology along with plate tectonics because Flat Earthers don’t follow the scientific method. The fact that scientists avoid universal truths and change their ideas as more evidence is uncovered shouldn’t be seen as meaning that the science is unsettled. Because of widespread scientific illiteracy, these arguments are used by those who wish to suppress science and misinform the general public.
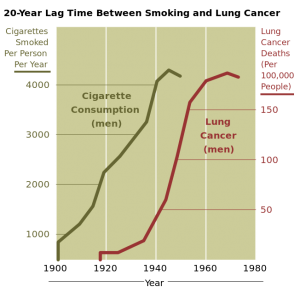
In a classic case of science denial, beginning in the 1960s and for the next three decades, the tobacco industry and its scientists used rhetorical arguments to deny a connection between tobacco usage and cancer. Once it became clear scientific studies overwhelmingly found that using tobacco dramatically increased a person’s likelihood of getting cancer, their next strategy was to create a sense of doubt about the science. The tobacco industry suggested the results were not yet fully understood and more study was needed. They used this doubt to lobby for delaying legislative action that would warn consumers of the potential health hazards [5; 7]. This same tactic is currently being employed by those who deny the significance of human involvement in climate change.
1.5 Evaluating Sources of Information

In the age of the internet, information is plentiful. Scientists, or anyone exploring scientific inquiry, must discern valid sources of information from pseudoscience and misinformation. This evaluation is especially important in scientific research because scientific knowledge is respected for its reliability [8]. Textbooks such as this one can aid this complex and crucial task. At its roots, quality information comes from the scientific method [9]. The application of the scientific method helps produce unbiased results. A valid inference or interpretation is based on objective evidence or data. Credible data and inferences are clearly labeled, separated, and differentiated. Anyone looking over the data can understand how the author’s conclusion was derived or come to an alternative conclusion. Scientific procedures are clearly defined so the investigation can be replicated to confirm the original results or expanded further to produce new results. These measures make a scientific inquiry valid and its use as a source reputable. Of course, substandard work occasionally slips through and retractions are published from time to time. An infamous article linking the MMR vaccine to autism appeared in the highly reputable journal Lancet in 1998. Journalists discovered the author had multiple conflicts of interest and fabricated data, and the article was retracted in 2010.
The same rigor should be applied to evaluating the publisher, ensuring the results reported come from an unbiased process [11]. The publisher should be easy to discover. Good publishers will show the latest papers in the journal and make their contact information and identification clear. Reputable journals show their peer review style. Some journal are predatory, where they use unexplained and unnecessary fees to submit and access journals. Reputable journals have recognizable editorial boards. Often, a reliable journal will associate with a trade, association, or recognized open-source initiative. In addition to methodology, data, and results, the authors of a study should be investigated. When looking into any research, the author(s) should be investigated [10]. An author’s credibility is based on multiple factors, such as having a degree in a relevant topic or being funded from an unbiased source.
One of the hallmarks of scientific research is peer review. Research should be transparent to peer review. This allows the scientific community to reproduce experimental results, correct and retract errors, and validate theories. This allows the reproduction of experimental results, corrections of errors, and proper justification of the research to experts.
Citation is not only imperative to avoid plagiarism, but also allows readers to investigate an author’s line of thought and conclusions. When reading scientific works, it is important to confirm the citations are from reputable scientific research. Most often, scientific citations are used to reference paraphrasing rather than quotes. The number of times a work is cited is said to measure of the influence an investigation has within the scientific community, although this technique is inherently biased [12].
We are at a point in time when a large amount of information is available to inform us on various topics, including science. All we have to do is type a question into Google and a multitude of answers appear. At some points, it can be overwhelming and often difficult to determine if a source is credible. It is particularly important to understand the credibility of a source when using it as a means to support your arguments and when making life decisions. When evaluating any source, you should ask yourself the 3 Ws:
- WHERE
Ask yourself where the source was published. Credible sources tend to be published in peer-reviewed scholarly journals or by a university press, professional society, or scientific publisher. This is because all of these sources of information are peer-reviewed, meaning they have been evaluated by experts in the field.
If the source is an online resource, you need to be particularly careful. It is not necessarily indicative of a poor resource, but it will depend on who published the information on the website. Generally, websites with a .gov or .edu will provide credible information. Conversely, a website like Wikipedia, though useful for quickly looking up information, is not a credible source to use because anyone can add and edit content, regardless of their expertise. Additionally, websites with a .com, .org, and other variations are often not credible sources based on biases associated with funding, a lack of people involved with the expertise to write the content, and a lack of peer-review. Also, keep in mind that there are some online journals (e.g. PlosOne) that will provide reputable, peer-reviewed journal articles.
- WHO
Ask yourself who the author is. Do they work at a University or another reputable institution? What have they written and do they have expertise in the content they have written? Additionally, you may want to assess anyone that the author, particularly in news articles, has decided to quote or use as a resource. As an example, there is an article on the site Slate.com about invasive boa constrictors in the Everglades. Two of the people Slate chose to interview for the article are associated with the Skunk Ape Museum in Florida. What is a skunk ape? Well, it is a mythical creature. Consequently, the credibility of the article is questionable. You can read a blog post on the issues with the article here and you can see the original article at this link.
Also, you should consider who the intended audience of the article is. If the intended audience is a scholarly one, there should be a clear bibliography included with the source you can consult. This becomes particularly important when secondary sources are providing information from a journal article or some other peer-reviewed source. It is a good idea to go take a look at the original resource to be sure that the authors are correctly providing information and not overstepping the conclusions that can be drawn from the paper.
- WHEN
Ask yourself when the article was written and if the content is outdated. Depending on the discipline, information can become outdated rapidly. You should look for additional texts on any given topic to be sure that the information provided is still relevant.
Test Yourself!
Go read this article (http://zapatopi.net/treeoctopus/) and use the 3 Ws to evaluate if it provides credible information.
For more information and practice, check out the Baloney Detection Kit
Summary
Science is a process, with no beginning and no end. Science is never finished because a full truth can never be known. However, science and the scientific method are the best way to understand the universe we live in. Scientists draw conclusions based on objective evidence; they consolidate these conclusions into unifying models. Scientists generate knowledge and information through their work, but science is not just a collection of facts. In addition, scientific knowledge is always generated based on the systematic gathering of objective data, but it is not always generated by lab-based experiments.