22 Chapter 22 The Respiratory System
By Krishnan Prabhakaran
Motivation.
COPD, or chronic obstructive pulmonary disease, is a progressive disease, which means it gets worse over time. With COPD, less air flows in and out of the airways, making it hard to breathe. In the United States, COPD affects more than 15 million adults, and many more do not know they have it. More than half of those diagnosed are women. COPD is a major cause of disability, and it is the fourth leading cause of death in the United States according to the Centers for Disease Control and Prevention (CDC).
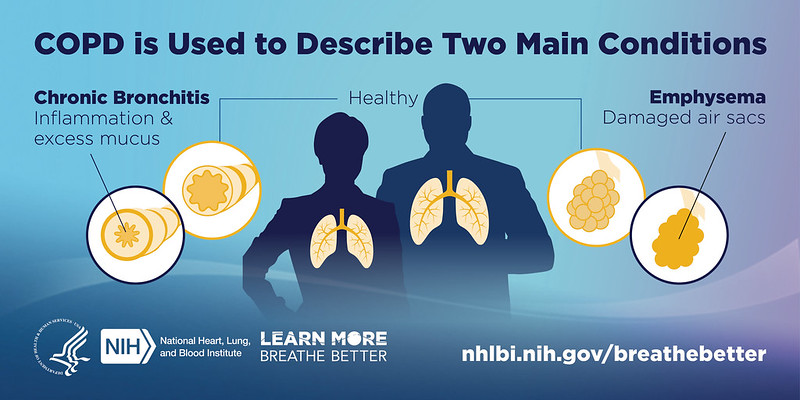
- Chronic (long-term) bronchitis (Figure 20.1, left) is caused by repeated or constant irritation and inflammation in the lining of the airways. Lots of thick mucus forms in the airways, making it hard to breathe.
- Emphysema (Figure 20.1, right) develops when there’s damage to the walls between many of the air sacs in the lungs. Normally, these sacs are elastic or stretchy. When you breathe in, each air sac fills up with air, like a small balloon. When you breathe out, the air sacs deflate, and the air goes out. In emphysema, it is harder for your lungs to move air out of your body.
COPD can cause coughing that produces large amounts of a slimy substance called mucus. It can also cause problems breathing, shortness of breath, chest tightness, and other symptoms. Symptoms of COPD often develop slowly but worsen over time, and they can limit your ability to do routine activities. Serious COPD may prevent you from doing even basic activities like walking, cooking, or taking care of yourself.
The good news is that COPD can often be prevented, mainly by not smoking. Cigarette smoking is the leading cause of COPD. Most people who have COPD smoke or used to smoke. However, up to 30% of people with COPD never smoked. A rare genetic condition called alpha-1 antitrypsin (AAT) deficiency can also cause the disease.
Other risk factors for COPD are:
- Exposure to certain gases or fumes in the workplace
- Exposure to heavy amounts of secondhand smoke and pollution
- Frequent use of a cooking fire without proper ventilation
Although there is no cure, treatments and lifestyle changes such as quitting smoking can help you feel better, stay more active, and slow the progress of the disease. You may also need oxygen therapy, pulmonary rehabilitation, or medicines to treat complications.
(Source: https://www.nhlbi.nih.gov/health/copd)
Learning Objectives
Upon completion of the work in this chapter students should be able to:
- Identify the major respiratory system structures on a cadaver specimen, model, or diagram, and state their function
- Identify tracheal or lung tissue features on a microscope slide relating structure to function
- Describe the role of muscle contraction and volume changes in the thorax in the mechanics of breathing.
- Describe the sounds heard with a stethoscope when breathing.
- Identify and explain the importance of various chemical and mechanical factors on modulating breathing rate
Background.
Anatomy of the Respiratory System
The major organs of the respiratory system function primarily to provide oxygen to body tissues for cellular respiration, to remove the waste product carbon dioxide, and to help maintain acid base balance. Portions of the respiratory system are also used for non-vital functions, such as sensing odors, speech production, and for straining, such as during childbirth or coughing
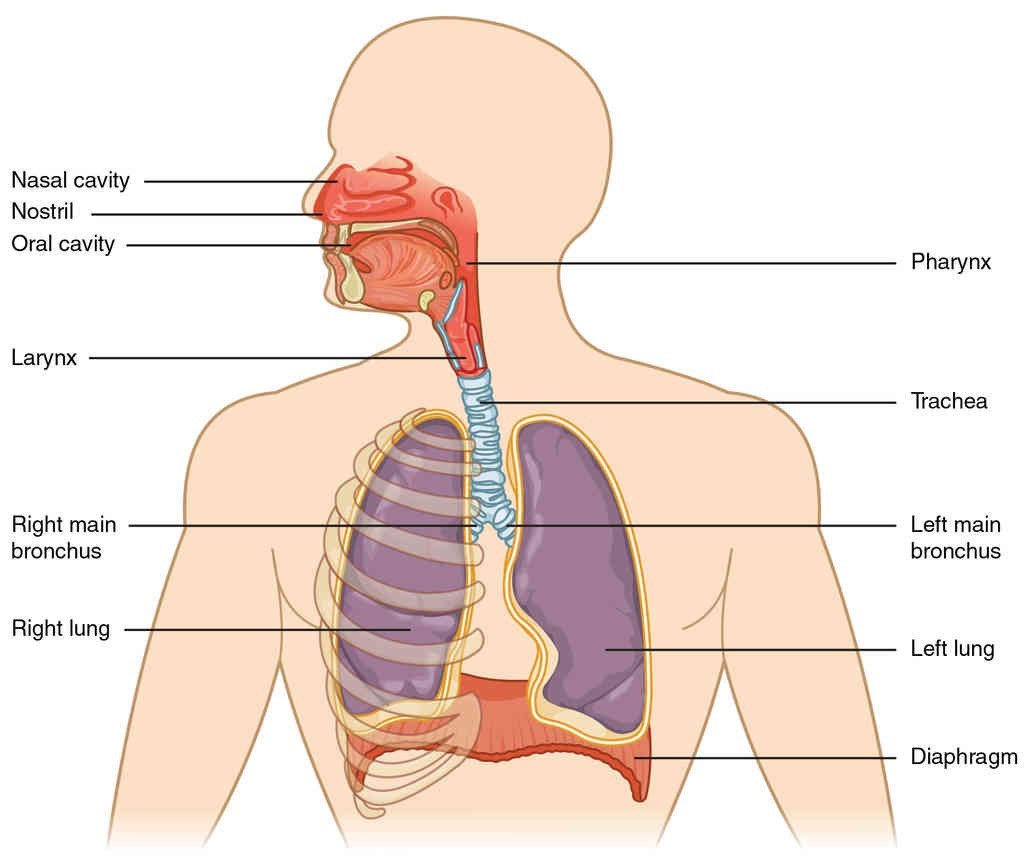
The major respiratory structures (Figure 22.2) extend from the nasal cavity to the diaphragm. Functionally, the respiratory system can be divided into a conducting zone and a respiratory zone. The conducting zone includes the organs and structures not directly involved in gas exchange, while gas exchange occurs solely in the respiratory zone. The major functions of the conducting zone are to provide a route for incoming and outgoing air, remove debris and pathogens from the incoming air, and to warm and humidify the incoming air. Several structures within the conducting zone perform other functions as well.
Nose and Nasal Cartilages
The major entrance and exit for the respiratory system is through the nose. When discussing the nose, it is helpful to divide it into two major sections: the external nose, and the nasal cavity or internal nose.
The external nose consists of the surface and skeletal structures that result in the outward appearance of the nose and contribute to its numerous functions (Figure 22.3). The root is the region of the nose located between the eyebrows, while the bridge is the part of the nose that connects the root to the rest of the nose. The dorsum nasi is the length of the nose, while the apex is the tip of the nose. On either side of the apex, the nostrils are formed by the alae
(singular = ala), which are cartilaginous structures that form the lateral sides of each naris (plural = nares), or nostril opening. Each external naris is protected by guard hairs.
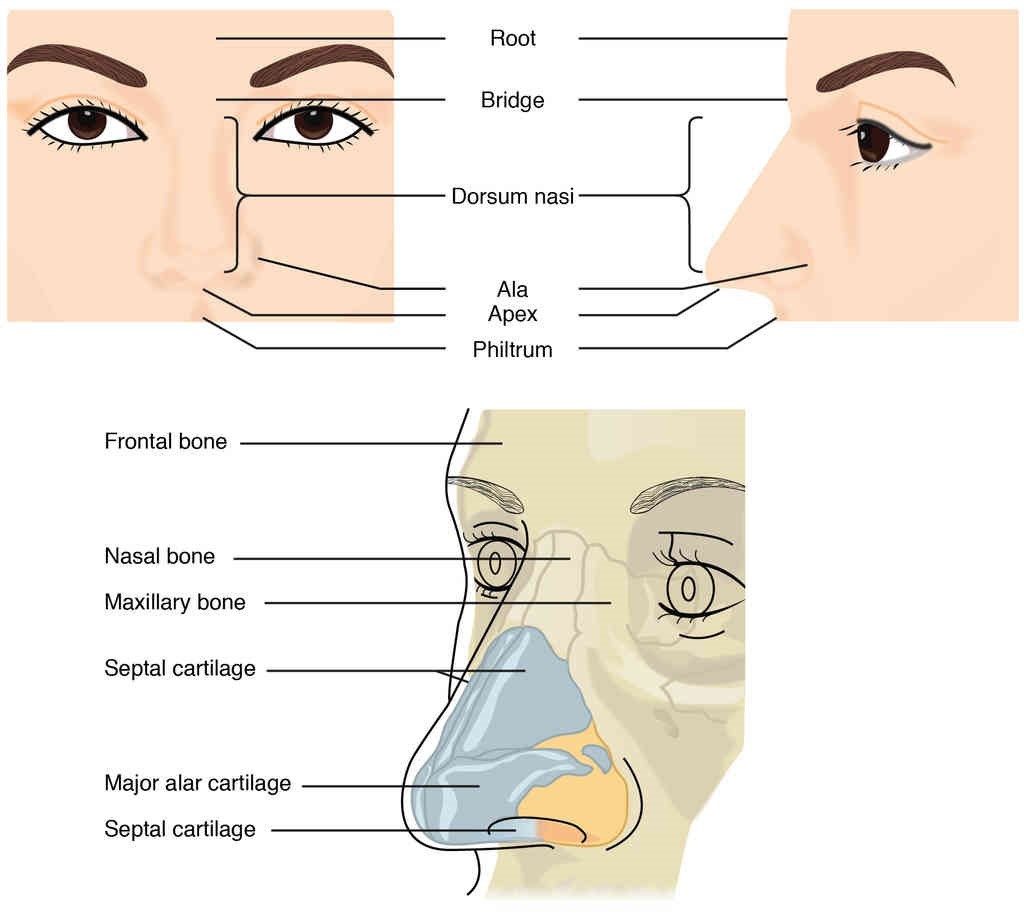
Underneath the thin skin of the nose are its skeletal features (see Figure 22.3, lower illustration). While the root and bridge of the nose consist of bone, the protruding portion of the nose is composed of cartilage. As a result, when looking at a skull, the nose is missing. The nasal bone is one of a pair of bones that lies under the root and bridge of the nose. The nasal bone articulates superiorly with the frontal bone and laterally with the maxillary bones. Septal cartilage is flexible hyaline cartilage connected to the nasal bone, forming the dorsum nasi. The alar cartilage makes up the apex of the nose and extends to surround each naris.
Internally, the nares open into the nasal vestibule, which is lined with stratified squamous epithelium. Behind the vestibule is the nasal cavity, which is separated into left and right sections by the nasal septum (Figure 3).The nasal septum is formed anteriorly by a portion of the septal cartilage (the flexible portion you can touch with your fingers) and posteriorly by the perpendicular plate of the ethmoid bone (a cranial bone located just posterior to the nasal bones) and the thin vomer bones (whose name refers to its plough shape). Each lateral wall of the nasal cavity has three bony projections, called the superior, middle, and inferior nasal conchae (singular = concha). Conchae serve to increase the surface area of the nasal cavity and to disrupt the flow of air as it enters into the nose, causing air to bounce along the epithelium, where it is cleaned and warmed. Each concha overlies a corresponding (superior, middle, inferior) meatus, or passageway that leads posteriorly away from the nasal cavity. The conchae and meatuses also conserve water and prevent dehydration of the nasal epithelium by trapping water during exhalation. The floor of the nasal cavity is composed of the palate. The hard palate at the anterior region of the nasal cavity is composed of bone, while the soft palate, at the posterior portion of the nasal cavity, consists of muscle tissue. Air exits the nasal cavities via the internal nares and moves into the pharynx (Figure 22.4).
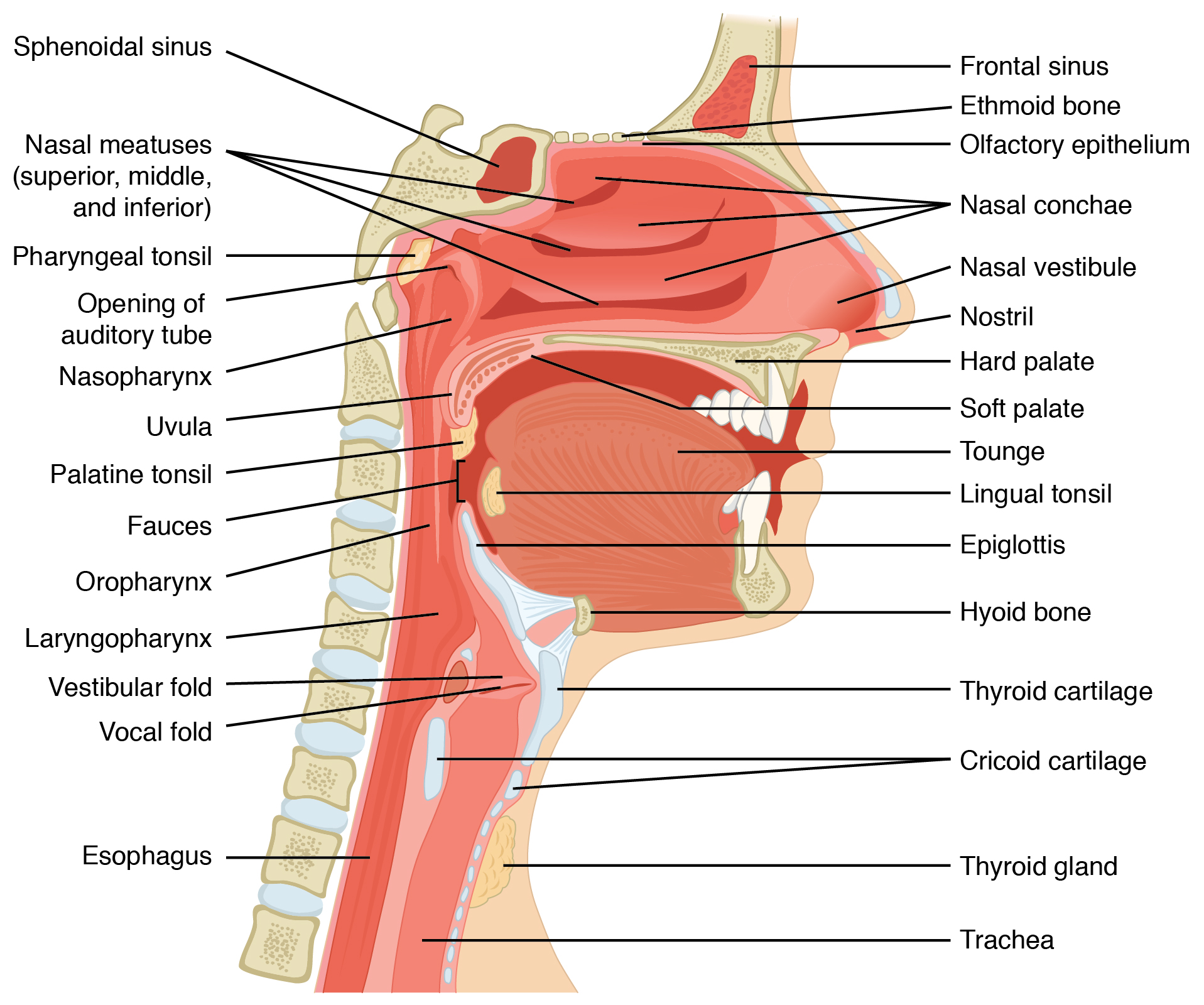
Behind the vestibule, the conchae, meatuses, and sinuses are lined by respiratory epithelium composed of pseudostratified ciliated columnar epithelium (Figure 22.5). The cilia of the respiratory epithelium help remove the mucus and debris from the nasal cavity with a constant beating motion, sweeping materials towards the throat so that it may be swallowed. This moist epithelium also functions to warm and humidify incoming air.
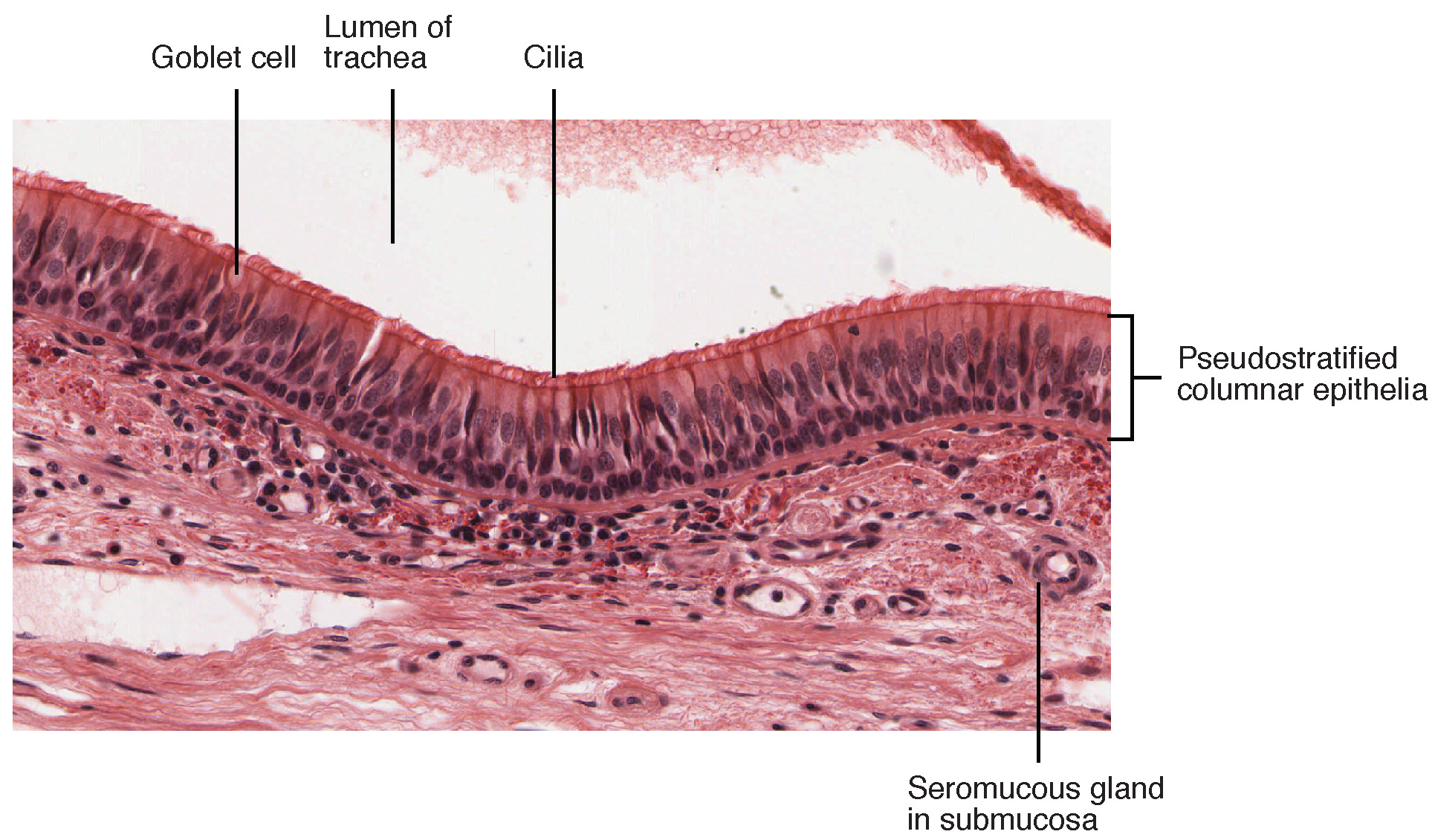
Pharynx
The pharynx is a tube that is formed by skeletal muscle and lined by a mucous membrane that is continuous with that of the nasal cavities (see Figure 22.4). The pharynx is divided into three major regions: the nasopharynx, the oropharynx, and the laryngopharynx (Figure 22.6).
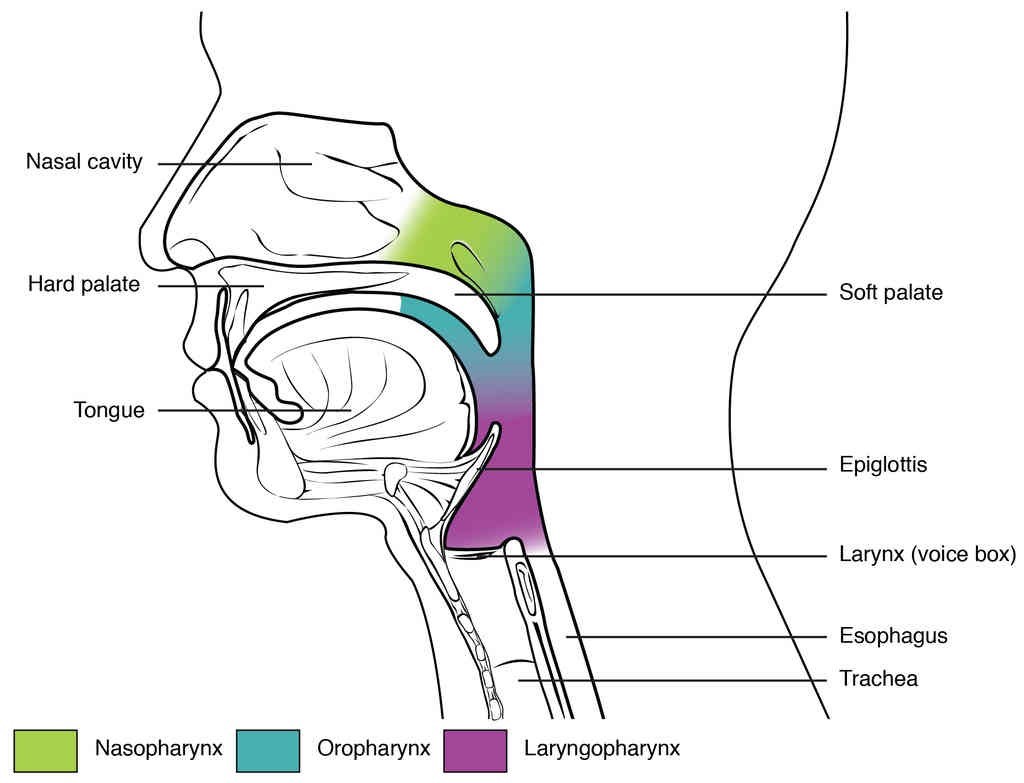
The uppermost nasopharynx sits directly posterior to the nasal cavity, and it serves only as an airway. The nasopharynx has two openings on the lateral walls though. These openings are the auditory (eustachian or pharyngotympanic) tubes, which connect the nasopharynx to each middle ear cavity. These connections are why colds often lead to ear infections. As the pharynx descends behind the oral cavity it becomes the oropharynx, which serves as a passageway for both air and food. The uvula is a small bulbous, teardrop-shaped structure located at the apex of the soft palate that partially separates the oral cavity from this region. Both the uvula and soft palate move like a pendulum during swallowing, swinging upward to close off the nasopharynx to prevent ingested materials from entering in to the nasal cavity. The most inferior portion of the pharynx is the laryngopharynx, which is located posterior to the larynx. It continues the route for ingested material and air until its inferior end, where the digestive and respiratory systems diverge. The stratified squamous epithelium of the oropharynx is continuous with the laryngopharynx. Anteriorly, the laryngopharynx opens into the larynx, whereas posteriorly, it enters the esophagus (Figure 22.6).
Larynx
The larynx is commonly known as the “voice box” because it is an important organ for sound production in humans. It is a cartilaginous structure oriented inferior to the laryngopharynx that connects the pharynx to the trachea and helps regulate the volume of air that enters and leaves the lungs (Figure 22.7). The larynx occurs about the level of the fourth through sixth cervical vertebrae and consists of a number of cartilages. Three large cartilage pieces—the thyroid cartilage (anterior), epiglottis (superior), and cricoid cartilage (inferior)—form the major structure of the larynx. The thyroid cartilage is the largest piece of cartilage that makes up the larynx. It is a shield-shaped structure made of hyaline cartilage. The thyroid cartilage contains the laryngeal prominence, or “Adam’s apple”, which tends to be more prominent in males due to the presence of increased testosterone levels. Inferior to the thyroid cartilage is the cricoid cartilage, which forms a ring. The cricoid cartilage also consists of hyaline cartilage and it appears relatively narrow when observed from the anterior, but increases in size at its posterior surface.
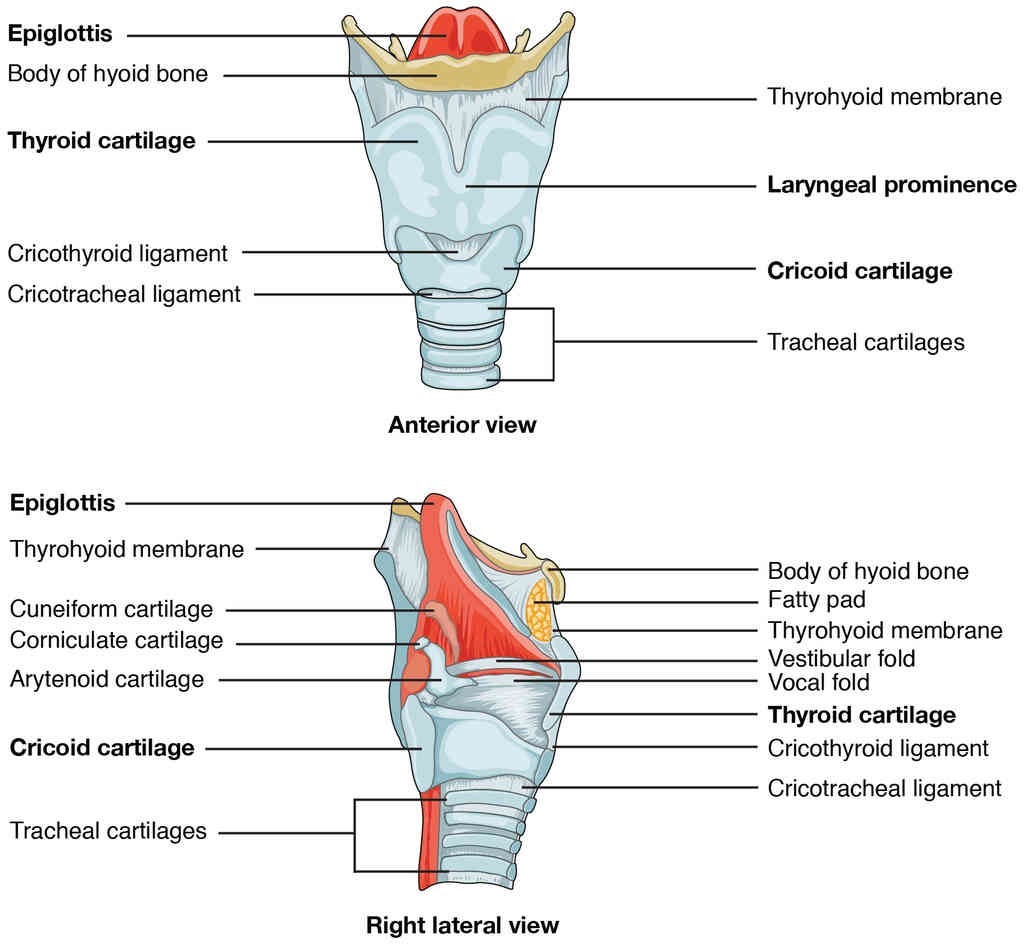
Three smaller, paired cartilages—the arytenoids, corniculates, and cuneiforms—attach to the epiglottis and the vocal cords and muscles that help move the vocal cords to produce speech (Figure 6). Superior to the cricoid cartilage in the posterior wall of the pharynx are the paired arytenoid cartilages. These cartilages attach to the posterior end of the vocal cords (vocal folds). Movement of the arytenoids pulls on the vocal cords, causing them to stretch and increase the pitch of the voice. This requires the contraction of intrinsic muscles attached to the arytenoid cartilages, while the vocal cords are held stationary by the thyroid cartilage anteriorly. Superior to the vocal cords are a folded pair of mucous membranes, known as the vestibular folds (false vocal cords) (Figure 22.8). At the posterior, superior edge of the larynx is the corniculate and cuneiform cartilages (Figure 22.7). Each of this is made from hyaline cartilage.
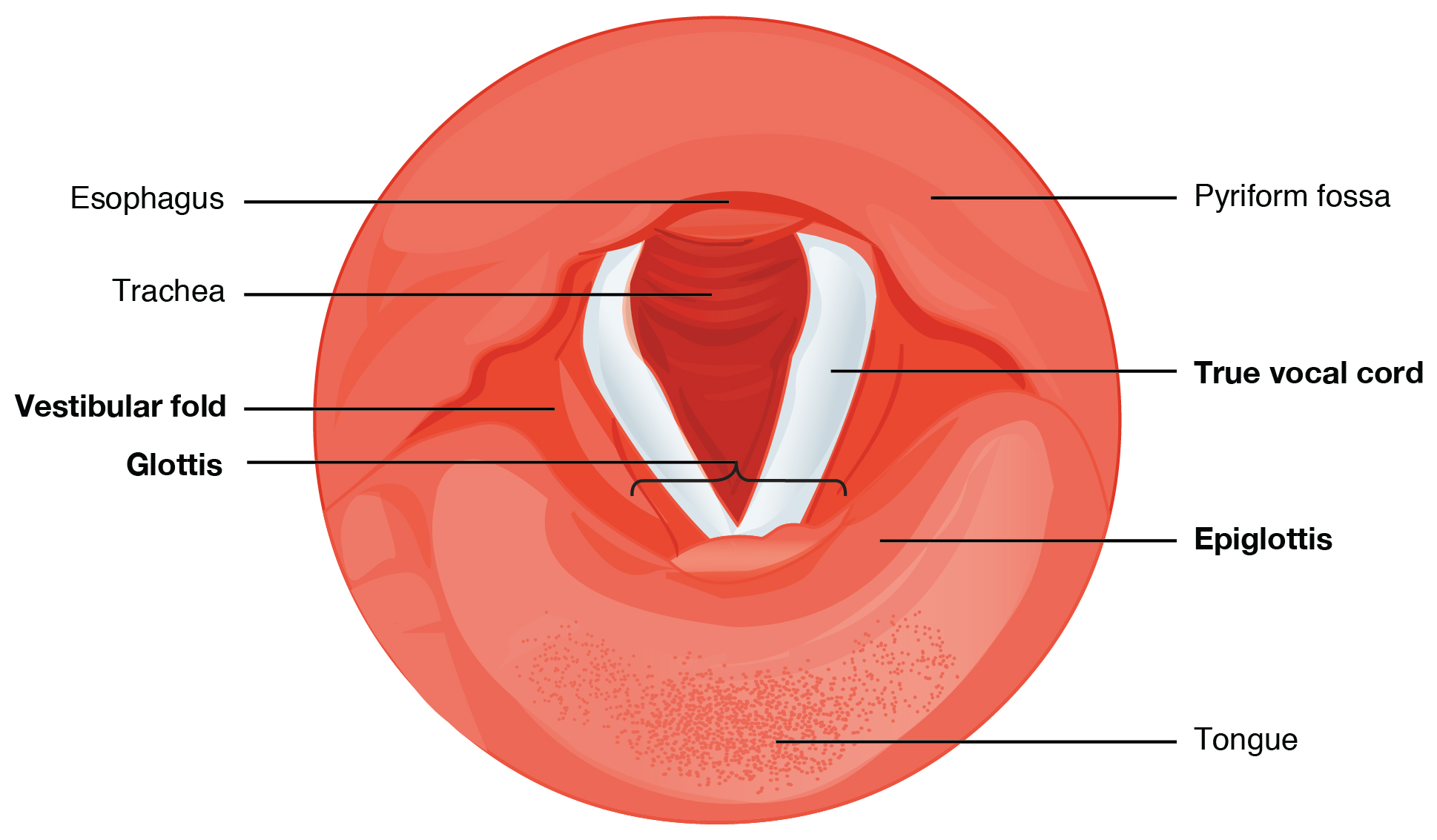
The most posterior cartilage of the larynx is the epiglottis, which is composed of elastic cartilage and mucous membrane. This cartilage is a very flexible piece of cartilage that covers the opening of the trachea (Figure 3). During swallowing, the epiglottis is pulled down and in to the “closed” position where the unattached end rests on the glottis. The glottis is composed of the vestibular folds, the true vocal cords, and the space between these folds (Figure 7).The act of swallowing causes the pharynx and larynx to lift upward, allowing the pharynx to expand and the epiglottis of the larynx to swing downward, closing the opening to the trachea. These movements produce a larger area for food to pass through, while preventing food and beverages from entering the trachea.
.
Trachea and Bronchi
The trachea is commonly known as the “windpipe” because it extends and carries air from the larynx toward the lungs (Figure 22.9a). The trachea is a straight tube whose lumen is kept open by 16 to 20 stacked, C-shaped tracheal cartilages. These cartilages are composed of hyaline cartilage and they are connected to one another by dense connective tissue. The trachea is also lined with respiratory epithelium, which is continuous with the larynx (Figure 22.9b). The esophagus borders the trachea posteriorly.
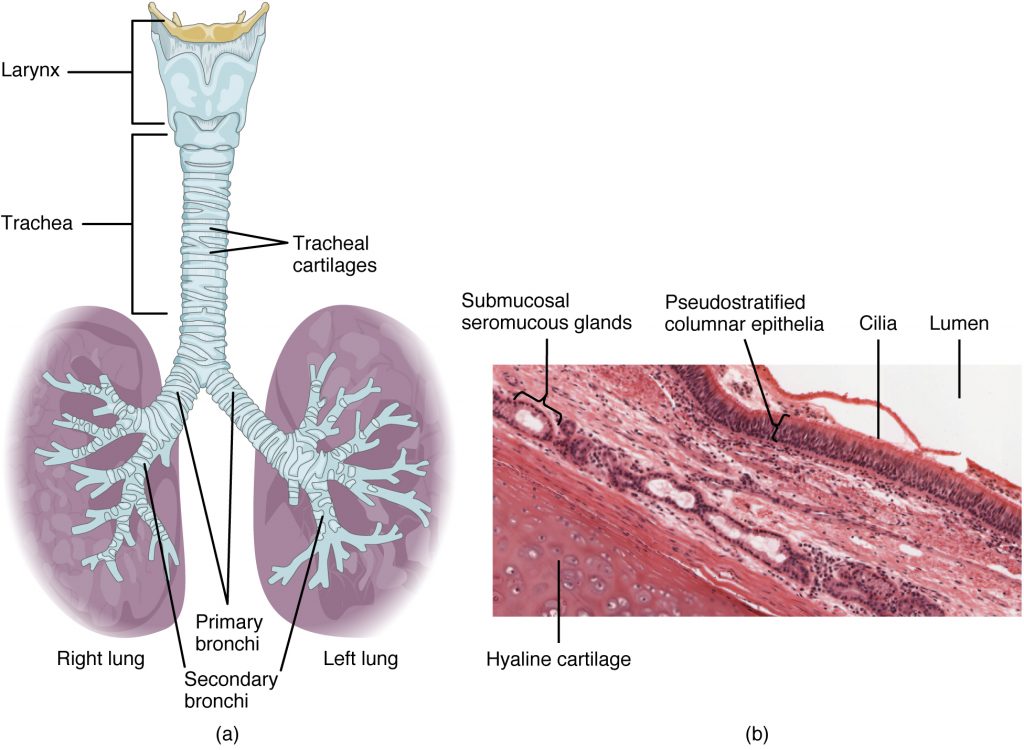
Bronchial Tree
At its most inferior end, the trachea branches into two tubes, which enter the lungs. These tubes are the right and left primary (main) bronchi. These bronchi are also lined by pseudostratified ciliated columnar epithelium containing mucus-producing goblet cells (Figure 22.9b). Rings of cartilage, similar to those of the trachea, support the structure of these bronchi and prevent their collapse. The primary bronchi enter the lungs at the hilum (Figure 22.10), a concave region where blood vessels, lymphatic vessels, and nerves also enter the lungs. Once inside the lungs, the main bronchi first divide into lobar (secondary) bronchi, which further divide to form the segmental bronchi (Figure 22.10). This extensive branching of the bronchi produces a structure called a bronchial tree (respiratory tree). The main function of the bronchi, like other conducting zone structures, is to provide a passageway for air to move into and out of each lung. In addition, the mucous membrane of these structures helps to trap debris and pathogens.
The bronchi continue to divide until they become the bronchioles, small respiratory tubes with smooth muscle in their walls, no cartilage, and an inner lining of respiratory epithelium (Figures 22.10 and 22.12). There are more than 1000 terminal bronchioles in each lung and the muscular wall can change the size of the tubing to increase or decrease airflow through the tube.
Lungs and Histology
From a gross perspective, the lungs are pyramid-shaped, paired organs that are connected to the trachea by the right and left bronchi. On the inferior surface, the lungs are bordered by the diaphragm (Figure 10). The right lung is shorter and wider than the left lung, and the left lung occupies a smaller volume than the right. The cardiac notch is an indentation on the surface of the left lung that allows space for the heart (Figure 9). The apex of the lung is the superior region, whereas the base is the opposite region near the diaphragm.
Figure 22.10. Gross anatomy of the lungs. Credit: OpenStax Anatomy and Physiology, license CC-BY-4.0
Each lung is composed of smaller units called lobes. The right lung consists of three lobes: the superior, middle, and inferior lobes. The left lung consists of two lobes: the superior and inferior lobes (Figure 22.10). Fissures separate these lobes from each other. In the right lung, the upper, horizontal fissure, separates the upper from the middle lobes while the lower, oblique fissure separates the inferior and middle lobes. Since the left lung only has a superior and inferior lobe, one oblique fissure is present, separating these two regions.
The lungs are enclosed by membranous sacs known as the pleurae, which are attached to the mediastinum. The pleurae consist of two layers. The visceral pleura is the layer that is superficial to the lungs, and extends into and lines the lung fissures (Figure 22.11). In contrast, the parietal pleura is the outer layer that connects to the thoracic wall, the mediastinum, and the diaphragm. The visceral and parietal pleurae connect to each other at the hilum and the pleural cavity is the space that sits between these layers.
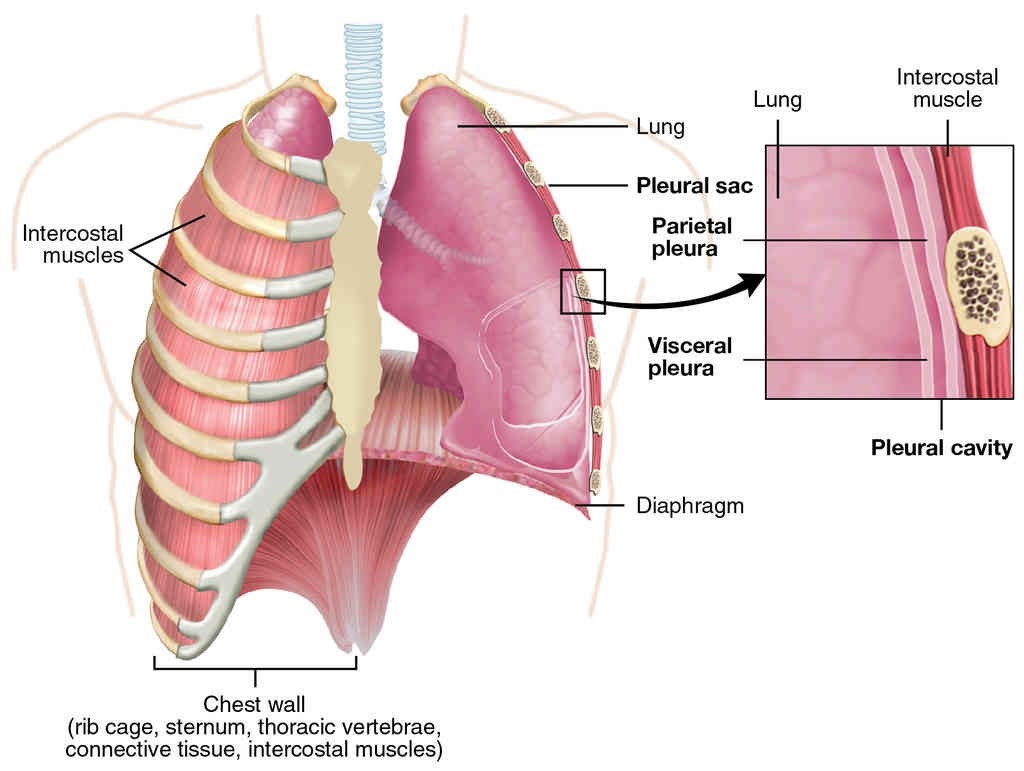
The pleurae perform two major functions: They produce pleural fluid and create cavities that separate the major organs. Pleural fluid is secreted by mesothelial cells from both pleural layers and it helps to reduce friction between the two layers to prevent trauma during breathing. It also creates surface tension that helps maintain the position of the lungs against the thoracic wall.
In contrast to the conducting zone, the respiratory zone includes structures that are directly involved in gas exchange. The respiratory zone begins where the terminal bronchioles join a respiratory bronchiole, the smallest type of bronchiole (Figure 22.12), which then leads to an alveolar duct. This passageway ultimately opens into a cluster of alveoli. An alveolus is one of the many small, grape-like sacs that are attached to each of the alveolar ducts. An alveolar sac is a cluster of many individual alveoli that are responsible for gas exchange.
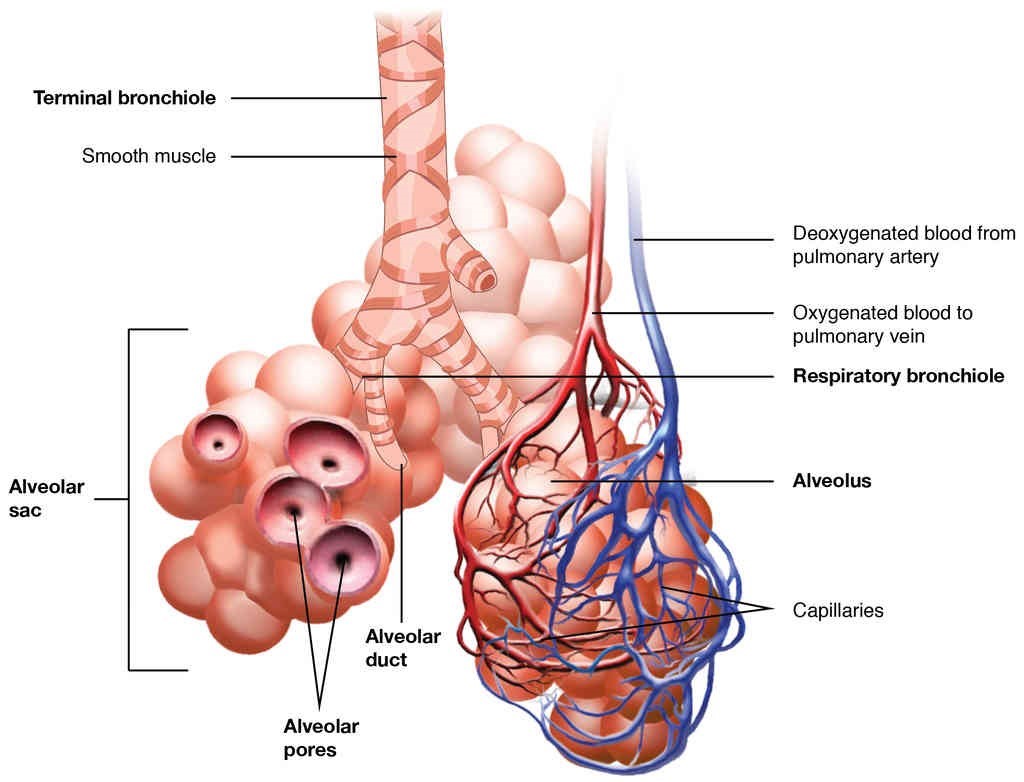
Each alveolus has elastic walls that allow it to stretch during air intake, which greatly increases the surface area available for gas exchange. Alveoli are connected to their neighbors by alveolar pores, which help maintain equal air pressure throughout the alveoli and lung (Figure 22.13).
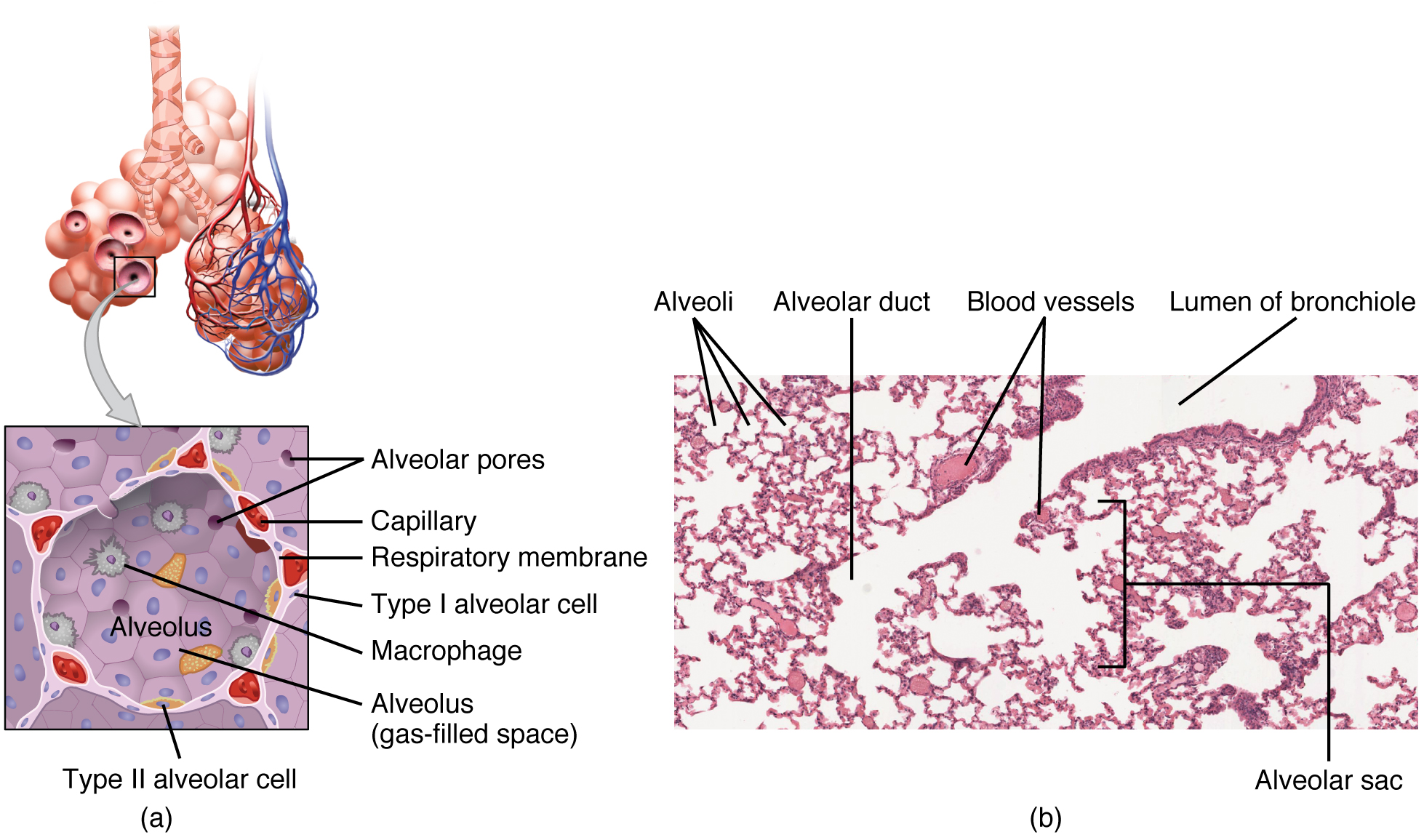
The alveolar wall consists of three major cell types: type I alveolar cells, type II alveolar cells, and alveolar macrophages. A type I alveolar cell is a squamous epithelial cell of the alveoli, which constitute up to 97 percent of the alveolar surface area. These cells are about 25 nm thick and are highly permeable to gases. Type II alveolar cells are interspersed among the type I cells and secrete pulmonary surfactant. This substance is composed of phospholipids and proteins that help to reduce the surface tension of the alveoli. Roaming around the alveolar wall are the alveolar macrophages, phagocytic cells of the immune system that removes debris and pathogens that have reached the alveoli.
Physiology of the Respiratory System
Mechanics of Breathing
Pulmonary ventilation is the act of breathing, which can be described as the movement of air into and out of the lungs. The major mechanisms that drive pulmonary ventilation are atmospheric pressure, the air pressure within the lungs, called intrapulmonary pressure, and the pressure within the pleural cavity, called intrapleural pressure. Atmospheric pressure is the amount of force that is exerted by gases in the air surrounding any given surface, such as the body. Atmospheric pressure can be expressed in terms of the unit atmosphere, abbreviated atm, or in millimeters of mercury (mm Hg). One atm is equal to 760 mm Hg, which is the atmospheric pressure at sea level. Intrapulmonary pressure is the pressure of the air within the lungs, which changes during the different phases of breathing. Intrapleural pressure is the pressure of the air within the pleural cavity, between the visceral and parietal pleurae. The difference in these pressures drives pulmonary ventilation because air flows down a pressure gradient, that is, air flows from an area of higher pressure to an area of lower pressure. Air flows into the lungs largely due to a difference in pressure; atmospheric pressure is greater than intrapulmonary pressure, and intrapulmonary pressure is greater than intrapleural pressure. Air flows out of the lungs based on the same principle; pressure within the lungs becomes greater than the atmospheric pressure.
Pulmonary ventilation comprises two major steps: inspiration and expiration, both of which are dependent upon the differences in pressure between the atmosphere and the lungs.
Inspiration is the process that causes air to enter the lungs while expiration is the process that causes air to leave the lungs (Figure 22.14). A respiratory cycle is one sequence of inspiration and expiration. In general, two muscle groups are used during normal inspiration: the diaphragm and the external intercostal muscles. Additional muscles can be used if a bigger breath is required though. When the diaphragm contracts, it moves inferiorly toward the abdominal cavity, creating a larger thoracic cavity and more space for the lungs. At the same time, contraction of the external intercostal muscles moves the ribs upward and outward, causing the rib cage to expand, which increases the volume of the thoracic cavity. Due to the adhesive force of the pleural fluid, the expansion of the thoracic cavity forces the lungs to stretch and expand as well. This increase in volume leads to a decrease in alveolar pressure, creating a pressure lower than atmospheric pressure. As a result, a pressure gradient is created that drives air into the lungs. Inspiration and expiration occur due to the expansion and contraction of the thoracic cavity, respectively.
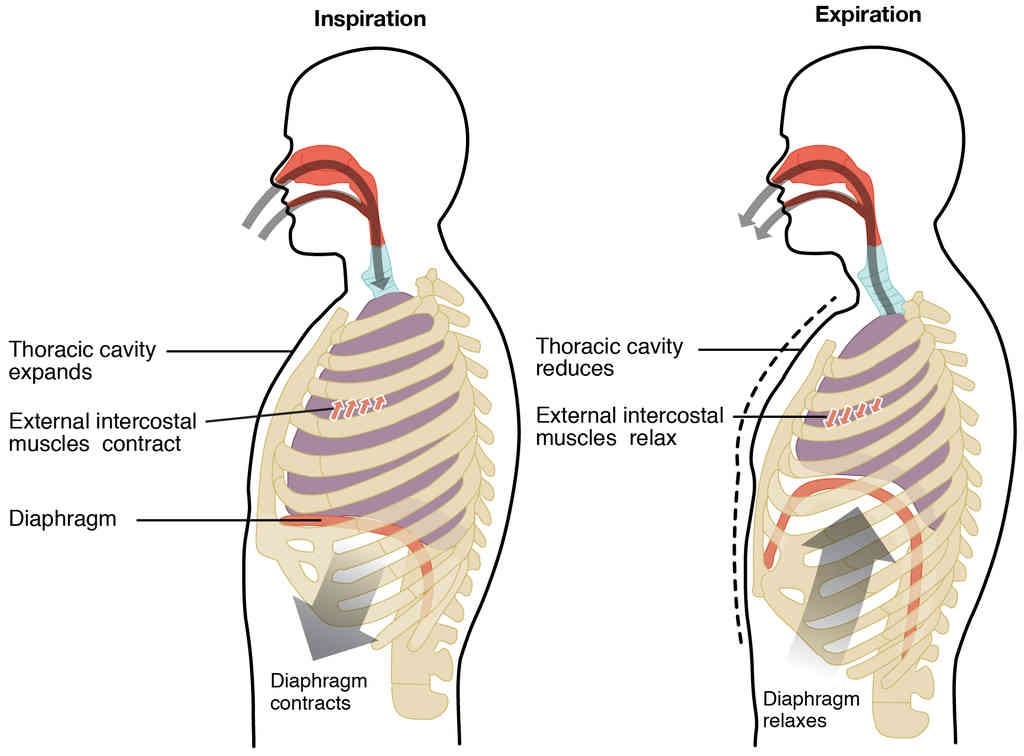
The process of normal expiration is passive, meaning that energy is not required to push air out of the lungs. Instead, the elasticity of the lung tissue causes the lungs to recoil, as the diaphragm and intercostal muscles relax following inspiration (Figure 22.2). In turn, the thoracic cavity and lungs decrease in volume, causing an increase in interpulmonary pressure. The interpulmonary pressure rises above atmospheric pressure, creating a pressure gradient that causes air to leave the lungs.
There are different types, or modes, of breathing that require a slightly different process to allow inspiration and expiration. Quiet breathing, also known as eupnea, is a mode of breathing that occurs at rest and does not require the cognitive thought of the individual. During quiet breathing, the diaphragm and external intercostals must contract. In contrast, forced breathing, also known as hyperpnea, is a mode of breathing that can occur during exercise or actions that require the active manipulation of breathing, such as singing. During forced breathing, inspiration and expiration both occur due to muscle contractions. In addition to the contraction of the diaphragm and intercostal muscles, other accessory muscles must also contract. During forced inspiration, muscles of the neck, including the scalenes, contract and lift the thoracic wall, increasing lung volume. During forced expiration, accessory muscles of the abdomen, including the obliques, contract, forcing abdominal organs upward against the diaphragm. This helps to push the diaphragm further into the thorax, pushing more air out. In addition, accessory muscles (primarily the internal intercostals) help to compress the rib cage, which also reduces the volume of the thoracic cavity.
Measurement of Respiratory Parameters
Gas exchange between air and blood occurs within the alveolar air sacs (Figure 22.15). The efficiency of gas exchange is dependent on ventilation. Cyclical breathing movement. Cyclical breathing movement alternately inflate and deflate the alveolar air sacs. Inspiration provides the alveoli with some fresh atmospheric air, and expiration removes some of the stale air, which has reduced oxygen and increased carbon dioxide concentrations.
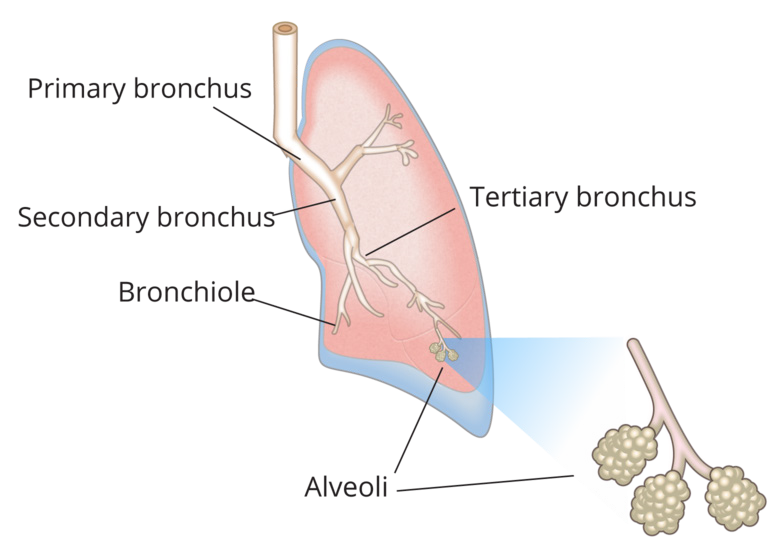
Pulmonary Volumes and Capacities
Spirometry allows many components of pulmonary function to be visualized, measured, and calculated. Respiration consists of repeated cycles of inspiration followed by expiration. During the respiratory cycle, a specific volume of air is drawn into and then expired from the lungs – the Tidal Volume (VT). In normal ventilation, the rate of breathing (breaths/minute) is approximately 15 respiratory cycles per minute. This value varies with the level of activity. The product of breaths/minute and VT is the Expired Minute Volume – the amount of air exhaled in one minute of breathing, which also changes according to the level of activity.
Note that the volume of air remaining in the lungs after a full expiration, residual volume (RV), cannot be measured by spirometry as it is impossible to exhale all the gas in the lungs. [There are specialized techniques to measure RV, but normally this volume is estimated from tables that predict RV based on age, sex, height and weight.]
The common lung volumes and capacities are shown in Figure 22.16 and Table 22.1, below. Note that the lung capacities are always the sum of at least two lung volumes, e.g., vital capacity (VC) is the sum of tidal volume (VT), expiratory reserve volume (ERV) and inspiratory reserve volume (IRV).
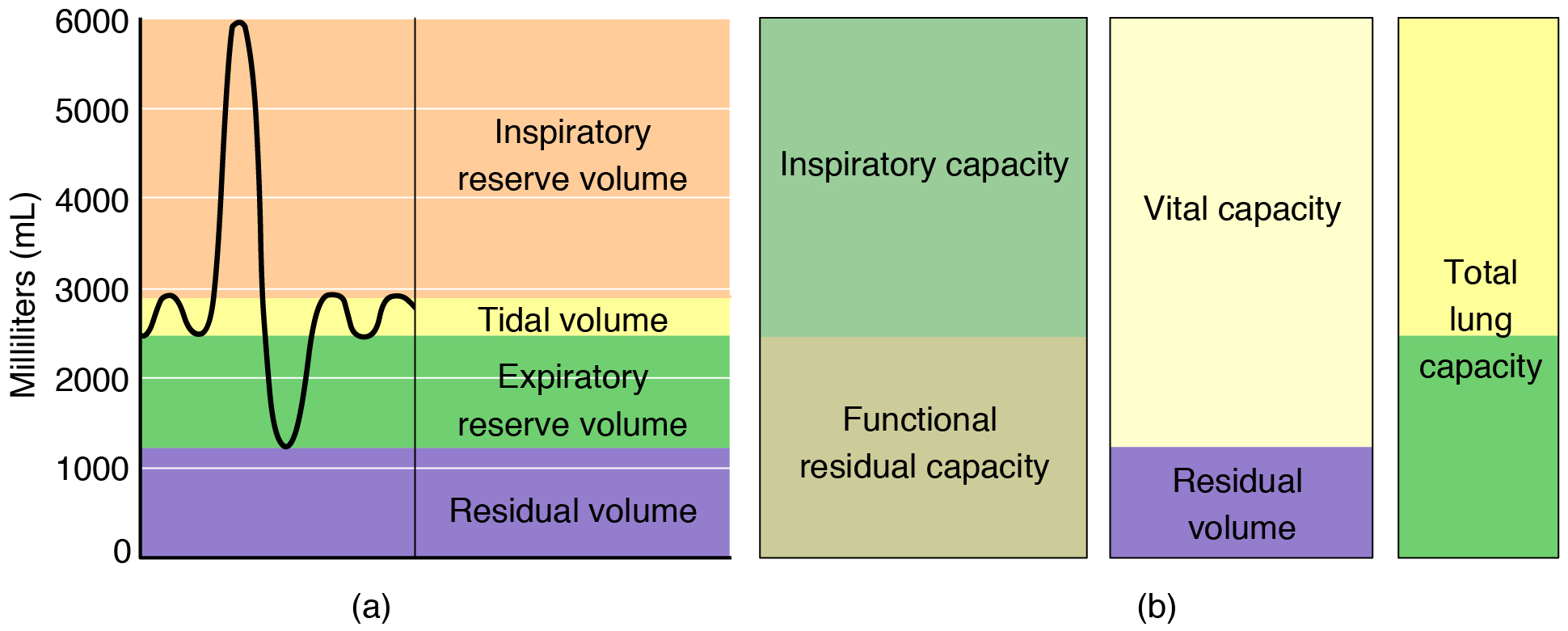
Lung volume measures:
- Tidal volume or VT: the volume breathed in and out in each breath.
- Inspiratory reserve volume or IRV: the maximum volume above the tidal volume that we can inhale into our lungs.
- Expiratory reserve volume or ERV: the maximum volume we can exhale from our lungs at the end of a normal breath.
- Residual volume or RV: the volume of air remaining in the lungs which is impossible for use to expire.
Lung capacity measures:
- Expiratory capacity (EC): the volume of air that we can expire after a normal inspiration and = V T + ERV.
- Functional residual capacity (FRC): the volume of air remaining in the lungs at the end of a normal expiration and = ERV + RV.
- Total lung capacity (TLC): all the air that it is possible for the lungs to contain and = RV + ERV + V T + IRV.
- Vital capacity (VC): all the air that can be expired following a maximal inhalation and = ERV + V T + IRV.
- Inspiratory capacity (IC): all the air breathed in during a maximal inhalation and = V T + IRV.
Respiratory Rate and Control of Respiration
Breathing usually occurs without thought, although at times you can consciously control it, such as when you swim under water, sing a song, or blow bubbles. The respiratory rate is the total number of breaths, or respiratory cycles, that occur each minute. Respiratory rate can be an important indicator of disease, as the rate may increase or decrease during an illness or in a disease condition. The respiratory rate is controlled by the respiratory center located within the medulla oblongata in the brain, which responds primarily to changes in carbon dioxide, oxygen, and pH levels in the blood.
The normal respiratory rate of a child decreases from birth to adolescence. A child under 1 year of age has a normal respiratory rate between 30 and 60 breaths per minute, but by the time a child is about 10 years old, the normal rate is closer to 18 to 30. By adolescence, the normal respiratory rate is similar to that of adults, 12 to 18 breaths per minute.
Dyspnea
Dyspnea refers to difficulty in breathing. What is difficult for one person is not necessarily so for another, so dyspnea has a psychological dimension. Perhaps the simplest view is to regard dyspnea as being a consequence of a mismatch between the afferent inputs that stimulate breathing (such as decreased PO2, increased PCO2, decreased pH, and activation of lung and chest wall receptors) and the efferent output to the muscles of respiration. That is, for whatever reason, breathing can not increase sufficiently to match the perceived central nervous system requirements, leading to feelings of distress and breathlessness.
Dyspnea may be acute or chronic. When people tell you that they are “breathless”, it is necessary to try to understand what they mean by this. Everyone gets breathless if they exercise vigorously. This is physiological and reversed rapidly when exercise finishes and should not be regarded as dyspnea. Illnesses that can be associated with the acute onset of dyspnea include pneumothorax, acute asthmatic attacks, pneumonia, myocardial infarction and rapidly developing heart failure. The major respiratory diseases associated with chronic dyspnea are COPD and restrictive lung disease. (Dyspnea section source material: ADInstruments NZ Limited 2019, license CC BY-SA)
Acid-Base Effects of the Respiratory Gases
The respiratory rate and the depth of inspiration are regulated by the medulla oblongata and pons; however, these regions of the brain do so in response to systemic stimuli. It is a dose response, positive-feedback relationship in which the greater the stimulus, the greater the response. Thus, increasing stimuli results in forced breathing. Multiple systemic factors are involved in stimulating the brain to produce pulmonary ventilation.
The major factor that stimulates the medulla and pons to produce changes in respiration is surprisingly not oxygen concentration, but rather the concentration of carbon dioxide in the blood. As you may recall, carbon dioxide is a waste product of cellular respiration and can be toxic. Concentrations of chemicals are sensed by chemoreceptors. A central chemoreceptor is one of the specialized receptors that are located in the brain and brainstem, whereas a peripheral chemoreceptor is one of these receptors located in the carotid arteries and aortic arch. Concentration changes in certain substances, such as carbon dioxide or hydrogen ions, stimulate these receptors, which in turn signal the respiration centers of the brain. In the case of carbon dioxide, as the concentration of CO2 in the blood increases, it readily diffuses across the blood-brain barrier, where it collects in the extracellular fluid. Increased carbon dioxide levels lead to increased levels of hydrogen ions, ultimately decreasing the pH of the blood. This increase in hydrogen ions in the brain triggers the central chemoreceptors to stimulate the respiratory centers to initiate contraction of the diaphragm and intercostal muscles. As a result, the rate and depth of respiration increase, allowing more carbon dioxide to be expelled, which brings more air into and out of the lungs. These actions promote a reduction in the blood levels of carbon dioxide, and therefore hydrogen ions. In contrast, low levels of carbon dioxide in the blood cause low levels of hydrogen ions in the brain and an increase in blood pH. These changes lead to a decrease in the rate and depth of pulmonary ventilation, producing shallow, slow breathing.
Another factor involved in influencing the respiratory activity of the brain is systemic arterial concentrations of hydrogen ions. Increasing carbon dioxide levels can lead to increased H+ levels, as mentioned above, as well as other metabolic activities, such as lactic acid accumulation after strenuous exercise. Peripheral chemoreceptors of the aortic arch and carotid arteries sense arterial levels of hydrogen ions. Removal of carbon dioxide from the blood helps to reduce hydrogen ions, thus increasing systemic pH. The peripheral chemoreceptors are also responsible for sensing large changes in blood oxygen levels. If blood oxygen levels become quite low—about 60 mm Hg or less—then peripheral chemoreceptors stimulate an increase in respiratory activity.
Pre-Laboratory Questions
- What is the role of the respiratory system, in terms of the overall function of the body?
- Which of the following statements regarding tidal volume (VT) is true?
- It is the volume breathed during forced breathing.
- It is the volume breathed in each breath.
- It is the volume breathed in each minute.
- It is unaffected by the frequency of breathing.
- Which of the following statements regarding the Expiratory Reserve Volume (ERV) is true?
- ERV is kept at a low volume so that the vast bulk of the alveolar gas can be replaced with fresh air during the next inspiration.
- ERV is the maximal amount of air that can be exhaled from the lungs after a normal expiration.
- ERV is very small and unimportant in normal respiration.
- Which of the following does Vital Capacity (VC) measure?
- The amount of gas that it is vital to retain in the respiratory system at the end of expiration.
- The maximum volume of gas in the respiratory system that can be exchanged with each breath.
- The volume of gas exchanged during normal breathing.
- Which of the following statements regarding Total Lung Capacity (TLC) is true?
- It increases as the frequency of breathing increases.
- It is a measure of the volume of gas in the respiratory system at the end of a maximal inspiration.
- It is constant in amount from person to person.
- In the respiratory system, what is the major difference between a volume and a capacity?
- A capacity is the sum of at least two volumes.
- A volume is the sum of at least two capacities.
Exercises
- Exercise 1 Study the anatomy and organization of the respiratory system
- Exercise 2 Pulmonary function measurements
Exercise 1 Study the anatomy and organization of the respiratory system
Required Materials
- Torso Model
- The Respiratory System Poster
- Respiratory System with Magnified Alveolus Model
- Half of the Human Head Model
- Median Section of the Head Model
- Human Larynx Model
- Smoker’s Lung Model
- Post-it notes
- Labeling tape
- Compound microscope
- Microscope lens cleaner
- Microscope lens solution
- Microscope immersion oil
- Slide of Mammal Trachea
Exercise 1A: Overview of the respiratory system
Procedure
- Look at the charts and models of the respiratory system for a general orientation and compare them to figure 22.1. Locate the following structures. Label these on the torso model or other models using post-it notes or labeling tape. Take a picture of your labeled model and insert the image below. Alternatively, you can sketch and label using the skeletal drawing to guide you or free drawing from models.
- Bronchus
- Nasal cavity
- Right lung
- Left lung
- Larynx
- Nose
- Trachea
- Pharynx
Exercise 1B: Nose and nasal cartilages
Procedure
- Examine a median section on a model or chart of the head and look for the nose, nasal cartilages, external nares (nostrils), and nasal septum.
- Examine the features identified in step 1 and compare them to what you see in Figures 22.3 and 22.4.
- Using the models and charts, locate the superior, middle, and inferior conchae in the nasal cavity.
- Identify where the nasal cavity ends, giving rise to two openings, the posterior nasal apertures, or choanae, which lead to the pharynx. Label all these nasal features using post-its or labeling tape, take a picture and insert your image below. Alternatively, you can sketch and label.
- The pharynx can be divided in to three regions based on location. Use the models and charts to find these regions.
- Examine the features from step 5 in Figures 22.4 and 22.6. Label the regions of pharynx using the post-it notes or labeling tape, take a picture and insert it below. Alternatively, you can sketch and label.
- The larynx is commonly known as the “voice box” because it is an important organ for sound production in humans. Using the models and charts, locate the prominent cartilages of the larynx; these include the thyroid cartilage, cricoid cartilage, and arytenoid cartilage. Locate the vocal cords (vocal folds), which attach to the anterior end of these cartilages.
- Examine the features from step 7 in Figures 22.7 and 22.8. Also use this figure to locate the vestibular folds, which are oriented superior to the vocal cords.
- Using the models and charts, locate the posterior structures of the larynx. These include the corniculate and cuneiform cartilages, the epiglottis, and the glottis.
- Examine the features from step 9 in Figure 22.7. Label these features of the larynx using post-it notes or labeling tape, take a picture and insert it below. Alternatively, you can sketch and label.
Exercise 1C: Trachea and bronchi
Procedure
- The trachea is a straight tube whose lumen is kept open by c-shaped tracheal cartilages. Examine these cartilages by running your fingers gently down the outside of your throat. Palpate the cartilage rings below the larynx.
- Locate the tracheal cartilages and the inferior carina in Figures 22.9 and 22.10. Label the trachea and tracheal features using post-it notes or labeling tape, take a picture of the labeled model and insert the image below. Alternatively, you can sketch and label.
- Obtain a prepared slide or a histological section of the trachea and find the tracheal cartilage, respiratory epithelium, and posterior tracheal membrane (absent in some slide preparations).
- Examine the features from step 3 in Figure 22.9.
- In the space provided below sketch what you observed at low and high magnification of the tracheal slide. Label the tracheal features listed in step 3.
- Using the provided models and charts, observe the extensive branching of the bronchi, which produce a structure called the bronchial tree.
- Examine the bronchial tree in Figures 22.9, 22.10 and 22.12. You should be able to identify the main bronchi, lobar bronchi, and segmental bronchi. Label these features on a model using post-it notes or labeling tape, take a picture and insert the image below. Alternatively, you can sketch and label.
Exercise 1D: Lungs
Procedure
- Look at the models or charts in the lab and identify the major features of the lungs. These include the superior lobe, middle lobe, and inferior lobe of the right lung and the superior lobe and inferior lobe of the left lung. Also identify the indentation of the left lung, the cardiac impression where the apex of the heart rests.
- Examine these lung features in Figure 22.10.
- Use Figure 22.11 to locate and identify the visceral and parietal pleura that surround the lungs. You should be able to identify the pleural cavity, which is the space that separates these two membranes. Label the lung structures mentioned in steps 1-3 on the models using post-it notes or labeling tape, take a picture and insert it in the space below. Alternatively, you can sketch and label these features below.
- On a model or chart identify the main bronchi. You should be able to see that the main bronchi continue to divide until they become bronchioles.
- The bronchioles continue to divide, leading to passageways known as the alveolar ducts, which branch into alveoli. Using the provided slide or image, locate the terminal bronchioles, respiratory bronchioles, alveolar ducts, and alveoli. Also examine these alveolar features in Figure 22.13.
- Using the models and charts, observe the alveolar sac, the cluster of alveoli located around the terminal end of the alveolar duct. Label the bronchiolar and alveolar structures mentioned in steps 4-6 using post-it notes or labeling tape, take a picture and insert it below. Alternatively, you can sketch and label these features in the space below.
- Observe the differences between a smoker’s lung and a normal lung using the Smoker’s Lung Model in the lab. Note the similarities and differences you observe in the anatomy of the healthy and diseased lungs shown. Write these down here:
Exercise 2 Pulmonary function measurements
Exercise 2A: Respiratory sounds
Required Materials
- Stethoscope
- Alcohol wipes
- Lab Partner
Procedure
- In this exercise you will listen to the breathing sound of an individual using a stethoscope.
- Find a partner to complete this activity with.
- Obtain a stethoscope.
- Clean the earpieces of the stethoscope with an alcohol wipe and let them dry.
- Make sure that the earpieces of the stethoscope point toward the anterior as you would insert them into your ear.
- Locate the larynx of your partner and place the diaphragm of the stethoscope just inferior to it.
- Listen for the sounds as your lab partner inhales and exhales. These are tracheal and bronchial sounds.
- Locate the triangle of auscultation, an area just medial to the inferior angle of the scapula. This is an ideal area for listening to sounds because fewer muscles cover this region of the thoracic cavity.
- Have your lab partner inhale and exhale deeply several times.
- Listen for a smooth flow of air into and out of the lungs. Wheezing or other rattling-like noises are indictors of congestion in the lungs.
- Record the observations of what you hear in the space below. Indicate the breathing condition of your lab partner:
Exercise 2B: Factors Influencing Rate and Depth of Respiration
In this exercise, you will first determine the breathing rate of an individual when they are at rest. Then, the volunteer will perform a series of activities to see how each one effects the rate of respiration over time.
Required Materials
- Stop watch (App on phone or iPad)
- Paper bag
- Lab partner
Part 1: Determining Resting (Quiet) Respiration Rate
Here, you will be determining the resting respiration rate of the subject. This will serve as a control to compare respiration rates to when you observe different activities.
Procedure
- Decide which partner will be the subject and which will bet the recorder. You may switch spots later, if time allows.
- The volunteer should sit comfortably and quietly in a chair for a minimum of one minute before recording begins.
- Using the stop watch, the recorder should then count the number of breaths taken by the subject over a 1-minute time period.
- Record this value in respirations/minute in the data Table 1 below.
Part 2: Factors Influencing Rate and Depth of Respiration
Procedure
- Again, have the test subject sit quietly in a chair to perform the first part of this activity. The same volunteer who you determined resting respiration rate for should also complete part 2.
- Have the volunteer preform each of the following activities listed in Table 1, below. The volunteer should perform each activity for a period of 1 minute.
- During each activity, record the subject’s breathing rate. Record this information in Table 1 as breaths/minute.
- Record any other observations that you think are pertinent in the Table 1.
Part 3: Determining Respiration Rate During Various Activities
Procedure
- You may choose a different volunteer to complete Part 3 of the activity. To begin part 3, have the test subject sit quietly in a chair to perform the first part of this activity.
- Have the volunteer preform each of the following activities.
Quiet Respiration
- Allow the subject to breathe normally for one minute.
- After this initial period, record the subject’s respiration rate (respirations/min.) during quiet inspiration. Enter the data in Table 2, below.
Deep Inhale
- Allow the subject to breathe normally for two minutes.
- After this initial period, have the subject deeply inhale and then hold their breath for as long as possible. Record (in seconds) how long the subject was able to hold their breath for. Enter this data in Table 2, below.
- As soon as the subject exhales, record the respiratory rate for several minutes (this time may vary) until a normal, resting breathing pattern returns. Also record how long it took (recovery time) for the subject’s breathing pattern to return to normal (in seconds).
- Enter this data in Table 2, below.
Forced Exhale
- Repeat the procedure for “Deep Inhale” above, but first the subject will inhale deeply, then exhale forcefully and completely, then hold their breath WITHOUT INHALING.
- Record the length of time (in seconds) that their breath was held and the respiratory rate until the subject has recovered.
- Enter this data in Table 2, below.
Hyperventilation
- Have the subject to hyperventilate (breath rapidly, about 1 breath/4 seconds) for approximately 30 seconds, then hold their breath for as long as possible.
- Record the length of time (in seconds) that their breath was held and the respiratory rate until the subject has recovered.
- Enter this data in Table 2, below.
Re-Breathing
- Have the subject breath into a paper bag for 3 minutes. WATCH CAREFULLY for unusual or unwanted reactions or behaviors.
- After this initial period, have the subject inhale as deeply as possible and then hold their breath for as long as possible. Record the length of time (in seconds) that their breath was held and the respiratory rate until the subject has recovered. Also record how long it took (recovery time) for the subject’s breathing pattern to return to normal (in seconds).
- Enter this data in Table 2, below.
Jogging In Place
- Have the subject jog in place (or run up and down the stairs) for 2 minutes.
- After this initial period, have the subject inhale as deeply as possible and then hold their breath for as long as possible. Record the length of time (in seconds) that their breath was held and the respiratory rate until the subject has recovered. Also record how long it took (recovery time) for the subject’s breathing pattern to return to normal (in seconds).
- Enter this data in Table 2, below.
Analysis
Table 1. Respiratory Rates During Various Activities
Tasks Performed | Observations and Rate of Breathing |
Quiet respiration | |
Talking | |
Yawning | |
Laughing | |
Standing | |
Concentrating | |
Swallowing water | |
Coughing | |
Lying down | |
Running in place |
Table 2. The Effect of Various Activities on Breath-Holding and Normal Recovery
Activity | Time Breath was held after activity (sec.) | Recovery time (sec.) | Respiratory rate during recovery (respirations/min) |
Quiet respiration | |||
Deep inhale and breath holding | |||
Deep inhale – forceful exhale and breath holding | |||
Hyperventilation | |||
Re-breathing (breathing into paper bag) | |||
Jogging in place |
Analysis Questions
- After inhaling and holding your breath, was your urge to inspire or exhale?
- After exhaling and holding your breath, was your urge to inspire or exhale?
- Explain your answers from questions 1 and 2.
- Explain the effect that hyperventilation has on respiratory rate and recovery. (HINT: Think about the gases that are found in the plasma during hyperventilation.)
Post-laboratory Questions
- Some of the nasal cartilages are made of hyaline cartilage. What functional adaptation does cartilage have over bone in making up the framework of the nose?
- The trachea branches into two tubes that go to the lungs. What are these tubes called?
- What small structure in the lung is the site of exchange of oxygen with the blood capillaries?
- Considering the differences that you observed between the normal lung and the smoker’s lung, how would these differences lead to functional differences in a nonsmoker versus a smoker?